INTRODUCTION
Obesity is associated with systemic low-grade chronic inflammation, which has been related to changes in iron metabolism 1,2,3,4,5. The inflammation condition is supported by increases in pro-inflammatory cytokines, such as tumor necrosis factor-alpha (TNF-α), interleukin-1 beta (IL-1β), interleukin-6 (IL-6), and from acute phase proteins, such as C-reactive protein 4,6.
The Roux-en-Y gastric bypass (RYGB) surgery employed in the treatment of morbid obesity affects iron supply to the organism through reduced food intake and/or through decreased intestinal absorption 7,8,9,10,11. Iron deficiency can also be aggravated by menstrual blood loss in reproductive-aged women 4,11,12,13.
Iron metabolism is affected by hepcidin, a hormone that promotes the inhibition of intestinal iron absorption, iron recycling by macrophages, and iron mobilization from the liver. In low-grade systemic inflammatory conditions, hepcidin is stimulated by inflammatory cytokine IL-6 14,15,16 and synthesized by hepatocytes and adipocytes 17.
Increased hepcidin concentrations in obesity have been linked to low-grade chronic inflammation and seems to contribute to reduced iron availability and mineral deficiencies in this condition 2,18,19. In this context, Tussing-Humphreys et al. 18 observed that decreases of lowgrade inflammation and hepcidin levels improved iron metabolism 6 months after restrictive bariatric surgery in premenopausal women.
Some studies have also indicated that serum ferritin levels tends to rise in obesity in response to proinflammatory cytokines 20,21 and can be used as both an indicator of iron stores and as an indirect inflammatory marker. After bariatric surgery, pronounced weight loss is accompanied by a reduction of low-grade inflammation, but a decrease in ferritin levels and anemia can also occur, mainly in premenopausal women 13,22.
Iron deficiency is the main cause of anemia after bariatric surgery, however, other micronutrient deficiencies (e.g., vitamin B12 and folic acid) can also influence iron metabolism 2,11,12,23. Bariatric surgery, especially RYGB surgery, induces a process of nutritional deficiencies that affect food intake and micronutrient absorption 23,24. With these conditions, micronutrient supplementation is indicated to maintain normalized iron levels and prevent anemia 2,11.
Changes in iron metabolism associated with obesity and after bariatric surgery, as with the effect of micronutrient supplementation, need to be better elucidated 25. Therefore, this study aimed to assess the effect of two micronutrient supplementation schemes on inflammation and iron metabolism in premenopausal women who had undergone RYGB surgery.
METHODS
SUBJECTS
This study included 45 women (aged 20-45 years; body mass index ≥ 35 kg/m2) in menacme and undergoing RYGB surgery. The exclusion criteria for participation in this study included: a) diabetes mellitus; b) anemia; c) hemoglobinopathies; d) thrombosis; e) infectious processes; f) hysterectomy; g) smoking; h) HIV positive; and i) the use of corticosteroids in the last six months. All participants signed an informed consent form after being informed about the research and procedures. This study was approved by the local Ethics Research Committee (protocol number: 3303-2009). The same medical staff performed the RYGB surgeries.
EXPERIMENTAL DESIGN
A prospective interventional study was conducted to assess the effect of two micronutrient supplementation schemes on iron metabolism and systemic inflammation markers. Sixty women on the waiting list for bariatric surgery were eligible and randomly allocated into one of two groups. Group 1 (n = 45) received daily micronutrient supplementation 30 days before surgery at a dose of 1 Recommended Dietary Allowance (RDA) (26) and 2 RDAs during the 6-month period after RYGB surgery. Group 2 (n = 15) received daily supplementation of 1 RDA during the 6-month period after surgery, according to the most commonly-used clinical practices adopted in Brazil. Additionally, we chose micronutrient supplementation instated of specific iron supplementation, because it is recommended according to clinical practice guidelines after bariatric surgery and specific deficiencies of micronutrients can influence iron metabolism. Completing all study procedures 34 subjects from group 1 and 11 from group 2, for a total of 45 subjects (75% of the subjects recruited). Anthropometric assessments, dietary intake, and blood samples were collected during baseline, after 30 days of micronutrient supplementation during the preoperative period, and at 6 months after the RYGB surgery in group 1. In group 2, the same assessments were collected at baseline and 6 months after the RYGB surgery.
BLOOD BIOCHEMICAL ANALYSIS
Twelve-hour fasting blood samples were collected by standard venipuncture in tubes containing ethylenediaminetetraacetic acid (EDTA) and without an anticoagulant. Hematocrit and hemoglobin were determined by flow cytometry. Serum analyses were conducted in automated equipment to determine: a) serum iron and total iron binding capacity (TIBC) by the calorimetric method; b) transferrin and high-sensitive C-reactive protein (hs-CRP) by the immunoturbidimetric method; and c) ferritin by the chemiluminescence method. Hepcidin and soluble transferrin receptor (sTfR) were determined by enzyme-linked immunosorbent assays (ELISA) using commercial kits (Human Hepcidin; USCN Life Science Inc., TX, USA) and Human sTfR (BioVendor, Candler, NC, USA). The TNF-, IL-10, and IL-6 were analyzed by chemiluminescence methods (Milliplex(r) MAP Human Cytokine; Millipore, Billerica, MA, USA). The reference values used were: hsCRP = 0.0-0.3 mg/dL; iron = 50.0-170.0 mg/dL; and hepcidin = 0.06-4.00 ng/mL (as suggested by the manufacturer [USCN Life Science Inc., TX, USA]).
Iron deficiency was identified when transferrin saturation index (TSI) values were less than 20%, calculated as: TSI = (serum iron/TIBC) x 100 (19,27). Anemia was defined as hemoglobin values < 12 g/dL, criteria established by the World Health Organization according to sex and age 29.
ANTHROPOMETRIC ASSESSMENT
Weight and height were assessed using a digital balance (Welmy, SP, Brazil) precise to within 100 g, and a stadiometer (Seca) precise to within 0.1 cm. Body mass index (BMI) was calculated as: body weight (kg) ÷ height squared (m2). The assessment procedures were conducted in accordance with Gibson 30.
MICRONUTRIENT INTAKE
A quantitative assessment was performed on nutritional intake from food records from three nonconsecutive days. The subjects were instructed to record all food and drink intake throughout the day. All subjects were trained by a dietitian, and the records were conferred to clarify any questions and to discuss the use of the micronutrient supplementation prescribed.
Food intake was recorded as household measures and converted into grams with the aid of a table of food intake in household measures 31. All data were tabulated in MS Excel software and quantitative data of nutrient intake were derived from the calculation based on information of food intake table (100 g) for the Brazilian population 32. The amount of micronutrient supplementation intake was added to the food intake. To remove personal variations, it was necessary to obtain intrapersonal (Sw 2) and interpersonal variances (Sb 2) using an analysis of variance (ANOVA), and the calculated adjusted average. In this study, vitamin intake was calculated for vitamins B6, B12, A, C, and folic acid, and mineral intake for iron, zinc, and copper.
MICRONUTRIENT SUPPLEMENTATION
The supplementation was manipulated in capsules before, and in powder after, RYGB surgery. The minerals presented in the formula were chelated into an amino acid (glycine). The micronutrient supplementation of 2 RDAs (26) was suggested to be given daily for RYGB surgery in two doses 11,22,23,24,25,26,27,28,29,30,31,32,33,34,35. Adequate Intake (AI) 26 was used when the RDA was not established for the micronutrient (except for calcium).
The amounts of vitamins and minerals provided in the 2 RDAs were: vitamin A (1,400 μg); vitamin D3 (cholecalciferol, 400 IU); vitamin E (-tocopherol; 30 mg); vitamin K (180 μg); vitamin C (150 mg); thiamine (2.2 mg); riboflavin (2.2 mg); nicotinamide (28 mg); pantothenic acid (10 mg); pyridoxine (2.6 mg); folic acid (800 μg); biotin (60 μg); cyanocobalamin (4.8 μg); magnesium (350 mg); calcium (500 mg); zinc (16 mg); copper (1,800 μg); chromium (50 μg); iron (36 mg); selenium (110 μg); manganese (3.6 mg); iodine (300 μg); silicon (10 μg); and vanadium (10 μg). A adequate intake (AI) 26 were used when RDA was not established for the micronutrient (except for calcium). The amounts of vitamins and minerals provided in 1 RDA were half the values described for 2 RDAs.
STATISTICAL ANALYSIS
Continuous variables were expressed as mean ± standard deviation, median, and minimum and maximum (min-max). Prior to statistical analyses, data normality were tested. For parametric data, comparisons were assessed by paired t-tests (two moments -pre to post), unpaired t-tests (between groups), and ANOVA (three moments) followed by Tukey's post hoc test. For non-parametric data, comparisons were assessed by the Wilcoxon test (two moments), the Mann-Whitney U test (between groups), and the Kruskal-Wallis test (three moments), followed by Dunn's post hoc test. The associations of continuous variables were analyzed using chi-square tests (2). The interaction between study variables, using the ferritin concentration as the dependent variable, was assessed by multivariate linear regression tests. The level of significance adopted was p < 0.05. Statistical analyses were performed using STATISTICA software 10 (StatSoft, Inc., Tulsa, OK, USA).
RESULTS
The groups investigated (group 1 [1 RDA pre and 2 RDA after] vs. group 2 [1 RDA after]) did not differ with regard to age (p = 0.874) and presence of comorbidities on admission into this study (p = 0.324). Among the investigated comorbidities, the frequencies identified were: hypertension (29%); dyslipidemia (31%); sleep apnea (11%); osteoarthritis (4.5%); and infertility (2.2%). The absence of comorbidities was 65% and the use of contraceptives was 29%.
At baseline assessments (for all subjects, n = 45), 95.5% and 91.0% had hepcidin and hs-CRP levels above reference values, respectively. Hypoferremia was identified in 2 subjects (4.5%), serum ferritin between 30-100 ng/dL in 27 subjects, and less than 30 ng/dL in 4 subjects (9.0%), however all hemoglobin values were ≥ 12 g/dL. Regarding iron deficiency (as determined by TSI values below 20%), it was observed in 10 subjects (22.2%) at baseline, 20.6% for group 1, and 27.3% for group 2 (Chi-square test, p = 0.643). The micronutrient supplementation schemes of 1 RDA before RYGB surgery resulted in reduction in iron deficiency for group 1 (5.9%). The supplementation schemes of 2 RDAs after RYGB surgery were associated with a reduction in iron deficiency for group 1 (2.9%, p = 0.032), but not for group 2 (27.3%, p = 0.100), who received micronutrient supplementation of only 1 RDA after RYGB surgery.
The results obtained before micronutrient supplementation (baseline), after preoperative supplementation (group 1), and after 6 months following the RYGB surgery, with regard to BMI and biochemical variables are show in table I. Group 1 had lower TIBC and higher TSI values compared to group 2, 6 months after the RYGB surgery. In the intragroup comparisons, both groups had decreased BMI and CRP-us 6 months after the RYGB surgery, and IL-6 interleukin decreases were evident only for group 1.
Table I Biochemical variables for groups 1 and 2 before and 6 months after RYGB surgery
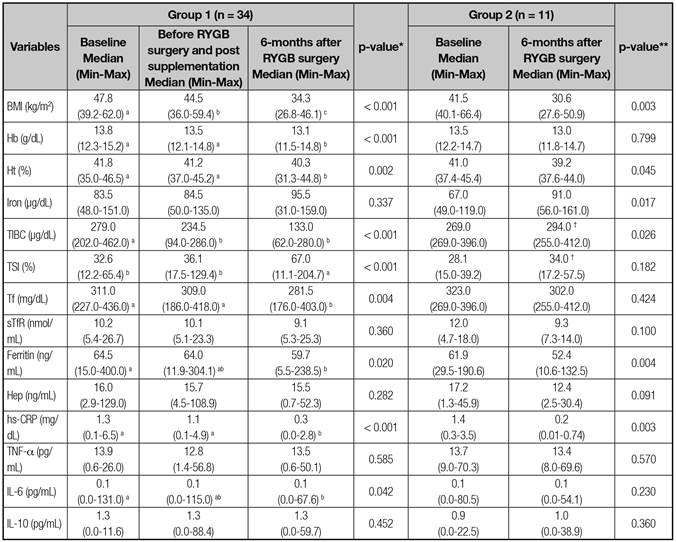
BMI: body mass index; Hb: hemoglobin; Ht: hematocrit; TIBC: total iron-binding capacity; TSI: transferrin saturation index; Tf: transferrin; sTfR: soluble transferrin receptor; Hep: hepcidin; hs-CRP: ultra-sensitive C-reactive protein; TNF-: tumor necrosis factor alpha; IL-6: interleukin-6; and IL-10: interleukin-10. *Friedman test for intra-group 1 comparisons, considering that variables marked with different letters on the same line were statistically different (p < 0.05). **Wilcoxon test for intra-group 2 comparisons. †Variables presenting statistical differences by the Mann-Whitney U test (p < 0.05) in the comparison between groups. Different letters on the same line are significantly different (p < 0.05).
For the variables related to iron metabolism, group 1 had reductions in TIBC, transferrin, and ferritin levels, and an increase in TSI values. For group 2, there were increases in TIBC and serum iron values, and a reduction of ferritin levels. Group 1 had a reduction in hemoglobin values, and both groups showed decreases in hematocrit levels 6 months after RYGB surgery (Table I). Six months after surgery, anemia was diagnosed in three subjects in group 1 and one subject in group 2, representing about 9% in both groups. It should be highlighted that there were four women who already had serum ferritin levels below 50 ng/dL prior to RYGB surgery (at baseline).
In the comparisons between groups, after micronutrient supplementation in the preoperative period, group 1 showed a significant difference compared with group 2 (preoperative period without supplementation) for TIBC (234.5 vs. 269.0 μg/dL for groups 1 and 2, respectively, p = 0.003) and TSI values (36.1 vs. 28.1% for groups 1 and 2, respectively, p = 0.036).
Regarding micronutrients, there was an increased intake after 6 months following RYGB surgery in both groups (except for vitamin B12, zinc and cooper for group 2). However, group 1 obtained higher values compared to group 2 due to the supplementation of 2 RDAs following surgery (Table II).
Table II Micronutrient intake for both groups before and 6 months after RYGB surgery

*Analysis of variance (ANOVA), followed by Tukey's post hoc test to compare intra-group 1 of the adjusted mean (± standard deviation), considering that the same variables marked with different letters on the same line presented statistical differences (p < 0.05). **Student´s t-tests to compare intra-group 2 of the adjusted mean (± standard deviation). †Variables that showed statistical differences by unpaired Student´s t-test (p < 0.05) in comparisons between groups. Different letters on the same line are significantly different (p < 0.05).
Multivariate linear regression analysis, using ferritin as a dependent variable, indicated that before and after RYGB surgery, the variable that best explained ferritin levels was hepcidin, followed by serum iron values and iron intake. Additionally, there was a significant influence of hs-CRP for determining ferritin levels during the perioperative period, and TSI in influencing ferritin levels 6 months after RYGB surgery (Table III).
DISCUSSION
This study investigated the influence of two micronutrient supplementation schemes of 1 RDA prior (for 1 month) and 2 RDAs after RYGB surgery (for 6 months) versus 1 RDA only after surgery (for 6 months). Both groups had significant reductions in body mass index (IMC), hs-CRP, IL-6, and ferritin levels after RYGB surgery. However, our data indicated that 1 RDA pre-surgery and 2 RDAs of micronutrient supplementation post-surgery was more efficient in controlling iron metabolism (increased TSI and reduced transferrin levels).
In general, the baseline analysis in our study revealed that subjects had alterations in iron metabolism (increased hepcidin levels and iron deficiency) and systemic inflammatory markers (hsCRP and IL-6). This data was consistent with morbid obesity as described in others studies 2,18,19,36. A previous study has shown a correlation between hepcidin and ferritin values prior to surgery and a reduction of inflammation 6 months after surgery was associated with hepcidin and sTfR reductions 18. In the current study, hepcidin determined ferritin values pre- and 6 months post-RYGB surgery, and with hs-CRP at baseline, showed a relationship between hepcidin and systemic low-grade inflammation in morbid obesity.
Hepcidin plays a key role in the regulation of iron metabolism, acting as a negative regulator of iron absorption in the intestine and stimulating iron retention in macrophages when higher systemic concentrations occur 37. The increase of serum iron values, as observed with group 2 after surgery (1 RDA micronutrient supplementation), indicates the effect of weight loss on improvements in iron status, and the role of hepcidin in this process. Group 2 had higher iron deficiency rates 6 months after RYGB surgery, probably due to a lower supplementation dosage, as indicated by lower TSI and higher TIBC values compared to group 1. Additionally, group 2 had a clear trend reduction in hepcidin values (28% vs. 2.5% for groups 2 and 1, respectively) during the same period (Table II). The reduction in hepcidin values occurs with iron deficiency to stimulate physiological pathways to elevate iron concentrations, thus, improving availability. Therefore, hepcidin probably had a lower percentage of reduction for group 1 due to the higher availability of iron via micronutrient supplementation (2 RDAs).
Ferritin is an important iron stores marker, but it also serves as an acute phase protein indicating systemic inflammation (8), and our multivariate regression analysis suggests this may be an accurate statement. Before surgery (at baseline), systemic low-grade inflammation influenced the increase of ferritin levels; however, before and after surgery, ferritin values were determined by serum iron levels, iron intake, and hepcidin. In obesity, increased ferritin levels are associated with low transferrin saturation and hypoferremia, but those with obesity do not seem to be more prone to anemia, compared to eutrophic subjects 25,38. Most of these changes are related to the anemia of inflammation, in which mechanisms are stimulated by proinflammatory cytokines and hepcidin 16.
In this study, at baseline assessments, only 2 subjects had low iron values, however, a low TSI was identified in 10 subjects (22.2%) and the majority (95.5%) of subjects had elevated hepcidin concentrations. Anemia caused by low-grade systemic inflammation or iron deficiency, occurs decrease of transferrin saturation 21, indicating that a functional iron deficiency may be associated with inflammation or a real mineral deficiency.
The effect of 1 RDA before and a 2 RDA micronutrient supplementation after RYGB surgery was effective in reducing iron deficiency (Table I). The number of subjects with iron deficiency was reduced (20.6% to 5.9% for baseline and pre-surgery, respectively) in accordance with TSI, after micronutrient supplementation during preoperative period of 1 RDA (group 1), indicating a real iron deficiency before surgery, since lowgrade systemic inflammation conditions persisted post initial supplementation.
Micronutrient supplementation of 2 RDAs after RYGB surgery was effective in maintaining TIBC, decreasing transferrin, and increasing TSI values. It is important to note that iron intake and TSI determined the increase of ferritin values 6 months after RYGB surgery when the analysis of both groups confirmed the influence of iron intake as the main marker of iron stores post-surgery in premenopausal women. The reduction of ferritin during the postoperative period increased the risk of anemia 13,23.
The current study indicated that low-grade inflammation with obesity contributed to changes in iron metabolism, however, this emphasizes the preoperative actual iron deficiency, as analyzed by the markers of TSI and ferritin levels. Of all subjects that completed the study (n = 45), 27 had ferritin levels between 30-100 ng/mL, suggesting an association of a functional and real iron deficiency, and 6 subjects had ferritin levels less than 30 ng/dL at baseline assessments, suggesting a real iron deficiency 21. Anemia was diagnosed in four subjects (~ 9%) within 6 months after RYGB surgery, with no difference between groups. However, all subjects had ferritin levels below 50 ng/dL, a value considered low for premenopausal women, and suggestive of the need for supplementation in the absence of anemia and presence of fatigue 28.
In an attempt to prevent decreases in hemoglobin and ferritin levels, and the development of anemia, which is a common outcome after RYGB surgery, specific recommendations are described in the literature 39. Aills et al. 33 recommend micronutrient supplementation at a dosage of 2 RDAs after malabsorptive surgery procedures, and in the specific case of iron, 36 mg per day. Recently, higher doses of iron (45-60 mg/day) have been suggested to prevent anemia in premenopausal women 40.
In our study, although there was a significant improvement in iron metabolism for group 1, the amount of iron supplementation before (1 RDA) and after (2 RDAs) RYGB surgery was insufficient to maintain postoperative erythropoiesis and a high number of subjects (premenopausal women) developed anemia. The decrease of ferritin levels occurred in both groups, showing a decrease of systemic inflammation levels, but also the beginning of an iron depletion process after RYGB surgery.
In the pre-surgical assessment, there are established serum iron levels, but no specification on minimum values for serum ferritin levels for submission to bariatric surgery 35,40. This may contribute to having patients with low iron stores, which may be more aggravating for premenopausal women and malabsorptive surgeries. Therefore, it is necessary to carefully assess iron metabolism and systemic inflammatory markers before RYGB surgery, and to establish a cut-off point for ferritin levels in premenopausal women since the depletion of iron stores associated with low nutritional demands after RYGB surgery culminate with premature anemia. It has been suggested that with a depletion of iron stores, as seen with ferritin levels of less than 50 ng/dL, the preoperative micronutrient supplementation and increase of iron intake after surgery is fundamental for premenopausal women. This was a prospective study that elucidated a small part of the metabolic and inflammatory events involved in morbid obesity, and in the context of RYGB surgery and iron metabolism. Other prospective and controlled studies with different micronutrient supplementation schemes will complement our findings. The limitation of the current study was the number of subjects, mainly for group 2, who were a sample of convenience patients cared for by the public health system.