INTRODUCTION
Hypoxia is a decrease in oxygen supply at the cellular level, which alters metabolism and energy production in aerobic organisms. In situations of normoxia (20.5-20.9 % ambient oxygen), there is a balanced ratio between oxygen supply and demand. However, in hypoxic conditions, the balance is altered, since there is a lower availability of oxygen (decrease in oxygen partial pressure) with an increase in the demand (1). High-intensity physical activity can cause something similar to an instantaneous hypoxia, as the increase in oxygen demand for a very short time period is higher than its availability. For this reason, highly intense and demanding exercise cannot be sustained for long compared with less intense efforts.
Hypoxic exposure in humans can be classified in different ways, but the main variable considered refers to the time that the individual stays in this condition. Therefore, according to the duration of the hypoxic conditions, hypoxia exposure can be classified in three other subgroups: short, long and intermittent exposures. Intermittent hypoxia (IH) is the main topic of the present review. IH is characterized by alternating cycles of hypoxia and normoxia. Scientific evidence has demonstrated that IH achieves similar adaptations than those obtained in chronic hypoxia, while avoiding the harmful side effects due to longer exposures.
“Living high and training low” is the first approach introduced in IH protocols (2). The goal was to stimulate an optimal adaptation to hypoxia, inducing circulatory changes to achieve a maximal aerobic capacity when training in normoxia. The hypoxia stimulus was applied during resting or recovering periods throughout the day. Nevertheless, hematological changes (increase in red blood cells) were dependent on the simulated altitude and time of exposure. Altitudes of 3,000-3,500 m are well tolerated together with hypoxic exposures of 12 h/day for a period of 15-18 days. Nevertheless, improvements in the VO2max of athletes were modest, and is still under debate whether this change was due to improvements in blood oxygen transport or to adaptations of muscle tissue. However, the studied protocols presented some methodological discrepancies, so it is difficult to conclude that sleeping at altitude or in hypoxia and training at sea level really improved sport performance (2).
Due to these discrepancies, IH protocols differentiate two additional possible situations: a) passive exposures to hypoxia; and b) active exposure to hypoxia, otherwise said, training in hypoxia but recovering or resting in normoxia. The first is more often used in the field of health and the second in sports performance, although recent evidence indicates that the latter may have applications in clinical protocols as well (3). In addition, these protocols must also consider if hypoxia exposure occurs in normobaric or in hypobaric (altitude) conditions. In this case, normobaric hypoxia is induced with tents or mask using different oxygen concentrations. This change allowed to combine training with competition demands, saving time in travels from high to low altitude regions. Nevertheless, the use of hypoxic tents (easily accessible for research than chambers) has favored mainly hypoxia protocols in normobaric conditions. This is the concept of IH (training in hypoxia and living in normoxia) that will be analyzed in more detail in the present narrative review.
INTERMITTENT HIPOXIA AND NUTRITION
Values of oxygen saturation at sea level are around 97-99 % in the human organism. Interestingly, women generally display one point higher than men, possibly as an adaptation to possible pregnancy. However, when oxygen saturation drops to 80-90 % and this situation is maintained (hypoxia), physiological adaptations occur. These include increases in respiratory and heart rate, in order to obtain more oxygen, due to the activation of the sympathetic-adrenal system. These adaptative changes cause increased metabolism and caloric expenditure. Therefore, in the first 3-7 days (acclimatization phase of the organism), this condition of caloric stimulation is accompanied by a drastic weight loss. This situation is very common in mountaineers who ascend high altitudes (base camps between 4,000 to 5,000 m), as well as in tourists who visit cities above 3,000 m altitude (4).
In addition, in a situation of hypoxia, active individuals have a strong dependence of glycolytic metabolism (5). Therefore, the physiological adaptation to hypoxia implies changes in energy metabolism displaying different energy priorities (3,6). At the same time, a decrease in appetite sensation under hypoxic conditions is observed (7). Increased leptin levels, among other hormones, seem to be involved in appetite modulation (8). Altogether, appetite control and increased glucose expenditure suggest that IH exposure could be a new strategy to consider in weight loss programs for obese people (3). On the other hand, this represents a limitation in athletes, since glucose consumption is further increased when compared to the same activity at sea level (9).
Finally, studies performed in humans and experimental animals indicate that hypoxia exposure can change the gut microbiota. However, interventions in IH conditions are scarce. In this context, a recent study with few participants indicated that IH exposure compared to participants in normoxia increased genera of anaerobic butyrate-producing bacteria. These results suggest that the changes in microbiota composition due to IH may affect glucose and lipid metabolism in the host (10).
INTERMITTENT HYPOXIA AND SPORT PERFORMANCE
In recent years, several studies have shown that IH training contributes to muscle adaptations that improve aerobic sport performance (11-16). However, other studies (17) present different results. This study (17) describes a slight increase in pedaling cadences in cycling (102 rpm at 200 m vs 107 rpm at 2,200 m). From 3,000 m, the cadence and pedaling power decreased by a rate of 7 % every 1,000 m. This suggests that above 3,000 m, specific competition training should not be planned. Altogether, it seems that optimal adaptations occur when hypoxic stimuli are properly controlled (18).
However, some authors suggest that the limiting factor for performance is anaerobic capacity, necessary for rhythm changes during the competition or for the sprint at the end of the race. In this context, training at high intensities is required to favor tolerance to high lactacidemias (19,20). However, this aspect has not been extensively studied in hypoxic conditions. Nevertheless, high intensity training performed in hypoxia seems to increase performance in several sports disciplines (21,22). Increase in glycolytic metabolism at submaximal efforts seems to be responsible for anaerobic improvements under hypoxic conditions (13,23). On the other hand, increase in the training load at sea level is the method used to improve anaerobic capacity, but often with the risk of overtraining due to the high intensity workout. However, in hypoxia there is an important activation of anaerobic metabolism without an increase in muscle load (20). Therefore, more studies designed to develop new intensive training methods performed in hypoxia could increase the adaptive possibilities of highly trained athletes, and therefore become more competitive.
In addition, Wingate tests performed in individuals after acute hypoxic exposure or after training in hypoxia indicated modest improvements in peak power as a result of changes in anaerobic metabolism (19,24). The underlying mechanism is yet unclear, although it seems that hypoxia increases cellular stress and metabolic changes to adapt to the high demands in anaerobic energy metabolism (23,25). However, the first organ affected by fatigue in anaerobiosis is the heart, not the skeletal muscle (25). Understanding the mechanism operating in this adaptation could be instrumental for an optimal planning of training programs. In this context, in many sport disciplines (i.e., swimming, rowing, skiing, cycling or endurance athletics), improving anaerobic metabolism is the limiting factor for performance at maximum oxygen consumption (20,22,23). The training theory states that physical demands must be greater to cause homeostatic imbalances, resulting in continuous adaptations (26). This aspect is more relevant in high-performance sport disciplines, where athletes are required to perform specific trainings that cause continuous imbalances and, at the same time, continuous improvements to increase physical performance (25,27).
In this context, increased expression of phosphofructokinase, a key enzyme in glycolysis, has been observed only in the case of high-intensity exercises in hypoxia (23,28). This leads to the hypothesis that the cycle of muscular hypoxia-hyperoxia during resistance strength exercises is insufficient to activate glycolytic metabolism, and additional stimuli are necessary. Therefore, training in IH appears as a new method to improve and optimize lactic capacity and power (29). The accumulated evidence supports a role for hypoxia-inducible factor-1 (HIF-1α) as the main factor responsible for hypoxia adaptation (28). In this sense, experiments in mice indicate that muscle damage and fatigue increase when muscle cells do not express HIF-Iα (30). Therefore, anaerobic muscle adaptations require the increased expression of HIF-Iα, which is activated when performing high-intensity training in hypoxic conditions. Hypoxia seems to suppress HIF-1α degradation by the proteasome system. Under these conditions, HIF-1α can translocate to the cell nucleus, where this transcription factor dimerizes with HIF-1β, forming the HIF heterodimer. The cAMP responsive element binding protein (CREBP) activates HIF heterodimer to bind to response elements in the promoters of target genes, including vascular endothelial growth factor (VEGF), erythropoietin (Epo), glucose transporters and glycolytic enzymes to support anaerobic metabolism (31). These metabolic and physiological responses allow an adaptation of the organism to the hypoxic conditions.
From all this information, a proposed protocol for training in IH is shown in table I. The protocol not only aims to improve sports performance, but also maintains body weight and cardiovascular health. To assess the importance of these stimuli for the improvement of cardiovascular function, the compensatory physiological mechanisms in response to hypoxia and their applications in health will be analyzed in the next section.
INTERMITTENT HYPOXIA IN HEALTH
One of the strategies to maintain good health of the athletes is by hypoxitherapy. Experimental studies have observed that hypoxitherapy can have multiple applications in pathologies of the respiratory (32), nervous (33,34), cardiovascular (35,36) and immune systems (37). These findings may be instrumental for sport disciplines where immune, cardiovascular and respiratory systems are challenged, such as extensive aerobic sports. Advantages can also be found in sports that compete in weight categories. Adjustments and changes in the different body functions (Table II) can be achieved with no health impacts. Therefore, it is important to understand the physiological compensatory mechanisms that occur to deal with acute and subacute situations of hypoxia, to understand the impact in the health of athletes.
Table II. Main compensatory physiological mechanisms in response to hypoxia.
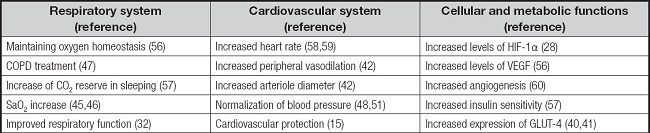
COPD: chronic obstructive pulmonary disease; GLUT-4: glucose transporter-4; HIF-1: hypoxia-inducible factor-1; SaO2: arterial oxygen saturation; VEGF: vascular endothelial growth factor.
HEALTH BENEFITS DUE TO PASSIVE EXPOSURE TO INTERMITTENT HYPOXIA
Sleeping in hypoxia results in supercompensation at the molecular level in order to increase the amount of oxygen supply to the organism. As previously indicated, one main actor in such changes is HIF-1α, increasing Epo expression as well as mitochondrial activity (38). In this context, Epo has been shown to produce neuro- and cardio-protective effects (33,35,36). In addition, stages at altitudes above 4,000 m lower blood glucose (39). Experiments performed in animal models indicate that this decrease in glycemia is due to increased GLUT-4 in the muscle membrane (40,41). This change could favor glucose uptake by the muscle and improve insulin sensitivity, suggesting that hypoxia may be a possible treatment for diabetes management. Nevertheless, future research needs to address these questions.
HEALTH BENEFITS DUE TO TRAINING IN INTERMITTENT HYPOXIA
IH training has several applications in certain pathophysiological situations, allowing to improve certain biological parameters without any relevant side effects (42,43). In addition, intense physical activity results in greater benefits in mitochondrial function, increasing physical capacity by 10 % in elders (44).
The ventilatory response to hypoxia increases in submaximal activities and induces a preconditioning to hypoxic states. The result is higher SaO2 during physical activity and sleeping when stages in hypoxic conditions (45,46). This would have direct applications to prepare mountaineers who want to go to Himalayan expeditions (above 8,000 m), as well as to perform high intensity training at altitudes. Moreover, it is effective in patients with asthma as well as with sleep apnea, by increasing hemoglobin levels and lung capacity (47).
In the cardiovascular system, IH training increases the diameter of arterioles (42) and lowers blood pressure in hypertensive patients (48,49), resulting in a hypotensive effect (50). Altogether, this suggests that IH training decreases cardiovascular risk (51-54).
Regarding the endogenous antioxidant defenses, training in IH increases superoxide dismutase expression while decreasing catalase expression in young people. However, catalase expression increases in elders under similar hypoxic conditions (55). Altogether, these results suggest that IH training could favor oxidative stress, particularly in young people, due to an increase in hydrogen peroxide produced by superoxide dismutase activity. Nevertheless, this response could be part of an adaptative response of the organism. In this context, other reports indicate that IH training decreases lipid peroxidation in healthy people, suggesting that IH training could potentiate antioxidant defenses (43). It has been reported that ROS induced in hypoxic conditions could release nuclear factor erythroid 2-related factor 2 (Nrf2) from Keap1 in the cytosol, avoiding its ubiquitin-proteasome degradation. Then, Nrf2 would migrate to the nucleus and bind to the coactivator Maf, recognizing the target genes coding for cellular antioxidants, such as superoxide dismutase, glutathione peroxidase and catalase, among others (31). In addition, oxidative stress is present in inflammatory processes associated with intense exercise performance. In this context, moderate or strenuous physical activity performed in IH modulates eosinophil- and platelet-related thrombosis, decreasing the secretion of the pro-inflammatory cytokine interleukin (IL)-1β and favoring the secretion of anti-inflammatory cytokines IL-6 and IL-10 (37).
Finally, in the context of metabolic disorders such as type 2 diabetes, physical activity and hypoxia possess additive effects in improving insulin sensitivity that seems to be mediated by increasing glucose transporters in trained muscle membranes, displaying higher levels when the intensity of muscle activity is high (40,41). Nevertheless, these last points need to be verified in more detail in humans training in IH. Altogether, IH training seems to induce muscular adaptations at the metabolic level that could be beneficial for the health of athletes as well as for the treatment of certain disorders.
INTERMITTENT HYPOXIA AND CONTROL OF BODY FAT MASS
Body weight reduction is constant in expeditions at high altitudes, affecting fat and muscle components (4). This response may be due to the thermoregulatory effect because of the intense cold at altitude (-5 to -40 °C), low energy intakes due to alteration in the digestive system function, and hypoxia, which causes a greater sympathetic-adrenal activation.
Studies carried out in Cerro Pasco (Peru) above 4,300 m in stages longer than one month documented losses of up to 15 % of body mass, as well as 40 % increased hematocrit levels and 100 % hypertrophy of the left ventricle (26). At the same time, anorexia occurred, contributing to body weight loss (4).
Serotonin is a key neurotransmitter in the control of food intake and appetite, which eventually would regulate body weight in the long term. In this context, administration of serotonin results in anorexia (60). In addition, exposure to hypoxia increases leptin levels as well as basal glycemia (7).
Studies in animal models corroborate all these observations (6,63). Altogether, these findings suggest that IH stimuli may be very useful for sports in which the limiting factor of performance is body weight, such as in athletes that compete in weight categories. In the health area, exposure to IH could be instrumental in the management of obesity. This is an open field for future research.
NEGATIVE ASPECTS OF HYPOXIA
When IH treatment is prescribed or during an ascent above 3,000 m, several key changes are noticed: a) respiratory rate increases; b) heart rate increases at rest and during activity; c) uncomfortable sleeping and insomnia; and d) high nervous stimulation. In addition, oxidative stress increases, particularly in altitudes of 5,500 m and during stages longer than four hours (64). These factors must be assessed when performing IH treatments.
CONCLUSIONS
Performing physical activity in hypoxia has adaptive advantages over normoxia training. Among them are the increase in the number of mitochondria, oxidative and lipolysis enzymes (14). Respiratory and cardiovascular adaptations also appear, with increased mitochondrial efficiency for ATP production, pH and lactate regulation, as well as improved muscular and physical performance (20,23). HIF-1α is responsible for all these adaptive changes that appear in hypoxic conditions, and participates in the activation of genes encoding for VEGF and Epo, which in turn have vasodilatory effects, promoting angiogenesis and cardiovascular protection.
In addition, exposure to hypoxia helps to lose body weight through increased sympathetic-adrenal stimulation, as well as decreasing appetite through the modulation of serotonin and leptin levels. Moreover, norepinephrine is increased one month after exposure to hypoxia, which would favor weight loss (8). Furthermore, the combination of hypoxia and physical activity allows to solve the loss of muscle mass, as it has been observed in expeditions at high altitudes and in animal models. Finally, many athletes risk their health by performing restrictive diets or high volumes of training to achieve very demanding challenges. IH stimuli allow these objectives to be attained while preserving the health of the athlete. Therefore, IH training would be very useful in set-up and pre-season periods, or in transition periods between seasons.
However, there are still some remaining questions to investigate. One of these is that continuous training above 3,000 m does not allow to maintain an adequate power (17). Therefore, it is necessary to optimize the training model at simulated altitudes of 3,000-4,500 m, to generate optimal adaptive responses at the metabolic and tissue level (14-16,19,20). All this implies changing the idea of altitude training and looking for more particular applications to train specific qualities in different season periods.