INTRODUCTION
The emergence of bioresorbable scaffold (BRS) started changing the landscape of percutaneous coronary intervention (PCI) and was considered by many the 4th revolution of interventional cardiology. The introduction of drug-eluting stents (DES) have significantly reduced the rates of angiographic restenosis and target lesion revascularization (TLR) compared with bare metal stents (BMS), and second-generation DES have further reduced the rates of stent thrombosis compared with both earlier generation DES and BMS [1]. Current DES are, although, impregnated with sophisticated platforms featuring biocompatible or biodegradable polymers they are not immune to long-term risk of vascular inflammation and stent thrombosis. This drives the impetus for manufacturing and developing BRS. Initial enthusiasm for the applications of BRS in the clinical scenarios has been mitigated by criticism of initial results in the near and long-term. Clinical evidence for BRS has been so far dominated by studies of the ®ABSORB scaffold (Abbott Vascular, Santa Clara, CA, USA), with thousands of patients treated in the setting of clinical registries and randomized trials. Literature on other BRS technologies, including devices available for clinical use is limited. This article reviews the outcomes previous and potentially upcoming BRS, focusing on the most recent stages of clinical development and future directions.
BENEFITS AND LIMITATIONS OF BIORESORBABLE SCAFFOLDS
BRS overcomes many of the limitations of metal stents. BRS can be made of natural substances that can naturally degrade overtime during the healing process post-PCI. These stents have been introduced to overcome the challenges that a nondegradable BMS and DES face. Thesechallenges include first that caging of the coronary artery by DES and permanently disrupting the fine endothelial layer of the artery with hindrance to the normal physiological conditions of the artery that leads to endothelial dysfunction [2]. Second, the altered flow of blood through a stented artery can also affect the endothelium function [2]. Third, as these stents are permanent implants warranting long term dual antiplatelet therapy. A lesion can be induced in the side branch in these patients due to reduced conformity to the vessel [3]. In addition, the long-term polymer exposure may result in chronic inflammation and hypersensitivity [4].
Once dissolves, BRS obviates the need for long-term antiplatelet therapy, removing the risk of local hypersensitivity and chronic inflammation [2]. It aims to leave a normal patent vessel with uninterrupted blood flow. The disappearance of the stent also has the potential to reinstate the vasoconstrictive properties of the vessel to sustain natural blood flow. However, the initial generation of BRS devices, principally the ®Absorb were hampered by increased rates of stent thrombosis compared with contemporary DES [5, 6]. This led to the withdrawal of the product from the market and a loss of trust in the technology. Newer-generation BRS devices utilizing different bioresorbable materials and featuring improved stent strut thick-ness may still provide a viable path to the "leave nothing behind" strategy.
CURRENT DEVICES
Contemporary BRS devices consist of either a metallic alloy (Magnesium or Iron alloy) or L-isomer poly-L-lactic acid (PLLA) polymeric platform, covered with a polymer and an anti-proliferative drug. Recently, Zn alloy (Zn-3Ag) has also been suggested to be a promising for BRS devices [7]. PLLA has lower tensile and mechanical strength, stiffness and ductility than metals, such as steel or Cobalt-Chromium (CoCr), used in contemporary DES. Therefore, scaffolds made from PLLA need to thicker struts and a wider strut profile than current DES for increasing tensile and radial strength [8]. Larger struts and higher percent surface area coverage, however, are associated with flow disturbances, impedance of endothelial coverage of the device, and implications for platelets activation and vascular healing [9]. Table 1 lists the BRS devices with CE-mark in Europe (®Absorb BVS, ®DESolve, ®ART Pure, ®Magmaris, and ®Fantom) along with their respective design properties.
ABSORB
The ®Absorb has been the most investigated BRS to date with promising signals for positive vascular remodeling at the lesion site, and improvement in vasomotion and endothelial function. Several prospective randomized trials (ABSORB II, ABSORB III, ABSORB CHINA, ABSORB JAPAN, TROFI II, and EVERBIO III) [10, 11, 12, 13, 14, 15, 16] were conducted which individually showed that cardiac death due to ®Absorb BVS device-related thrombosis and clinically driven target lesion revascularization (TLR) were statistically similar to those with contemporary cobalt chromium everolimus-eluting stent (CoCr-EES) [Abbott Vascular, Santa Clara, CA, USA)]. However numerically the rates for definite or probable scaffold thrombosis were consistently higher for ®Absorb BVS as compared to CoCr-EES. The worse outcome could have been due to large strut thickness of the scaffold resulting in local hemodynamic alteration, platelet activation and increased thrombogenicity.
In addition, the breakdown products and/or the extracellular matrix replacing the strut void may induce inflammation and thrombogenicity. Finally, underexpansion and malapposition may be relatively frequent due to the inherent physical properties leading to greater likelihood of acute recoil and strut fracture [17]. However, this provided some valuable insight into BRS deployment techniques. Optimal deployment techniques that aimed at reducing inadequate vessel sizing, malposition, and scaffold underexpansion appeared, in smaller sub-studies, to reduce the rates of scaffold thrombosis, and the "PSP" technique (pre-dilation, proper sizing, and post-dilation) emerged that could influence target lesion failure (TLF) and stent thrombosis. Pre-dilation (non-compliant balloon to reference vessel diameter ratio ≥1:1), vessel size selection (reference vessel diameter ≥2.25 mm and ≤3.75 mm), and post-dilation (with a noncompliant balloon at ≥18 atm and larger than the nominal scaffold diameter, but not by >0.5 mm larger) in all BVS-treated lesions were performed in only 59.2%, 81.6%, and 12.4% of patients, respectively.
DESOLVE
®DESolve (Elixir) is novolimus-eluting BRS with PLLA polymer backbone. The ®DESolve polymer scaffold is characterized by early degradation (>90% at 6 months) and resorption (∼70% bioresorption at 12 months) and provides a larger range of expansion and unique self-correction feature that can resolve minor malapposition to the vessel wall [18]. Neither randomized controlled trials nor robust data exist comparing ®DESolve to ®Absorb BVS, other BRS devices, or CoCr metallic DES. However, the propensity matched analysis demonstrated no difference in clinic performance of ®DESolve compared to ®Absorb BVS at 1 year [19]. Serial intravascular ultrasound (IVUS) has revealed an increase in the device and lumen dimensions between baseline and 6 months, with no detectable footprints of the scaffold at 18 months [20]. At 5-year clinical and imaging follow-up of the DESolve Nx clinical study [21] no definite scaffold thrombosis was reported but cardiac deaths were 2.5% and TLR rate was 4.1% (Table 2).
Table 2. Clinical performance measures of ®DESolve, ®ART Pure, ®Magmaris, and ®Fantom BRS devices
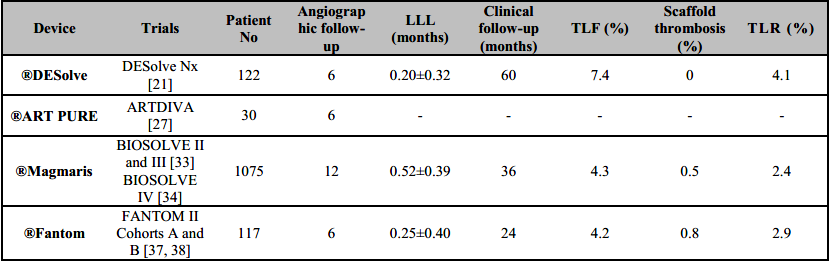
LLL: Late lumen loss; TLF: Target lesion failure; TLR: target lesion vascularization.
The ®DESolve PMCF [22] study showed 1% definite scaffold thrombosis, 3% TLR rate, 1% myocardial infarction (MI) rate, and no cardiac deaths at 12 months. Registry data has documented low MACE rates on long-term follow-up [23]. Early data on next generation ®DESolve Cx is encouraging, with no reported ischemic events or device thrombosis at 12 months follow-up [24]. It has been hypothesized that it may either contribute to the reduced risk of malfunction of the stent immediately after implantation or may prove to be a beneficial feature in acute myocardial infarction, where the stent may be undersized. However, there is no data to support this concept [25]. The new model promises biodegradation in the first year to the extent as much as 95% with the assumption of full resorption of the stent up to 2 years [25, 26]. After this period, the polymer is replaced with a loose net mainly composed of proteoglycan, followed by a new connective tissue [25]. ®DESolve scaffolds differ, therefore, from ®Absorb stents due to the properties of self-expansion and increased tolerance to excessive stretching.
ART PURE
®ART Pure BRS device consists of a PDLLA polymer backbone with no eluted drug and a strut thickness of 170 µm. This design might confer a rapid degradation of the polymer, rapid endothelial coverage of the device, and potentially reduced risk of late device thrombosis. However, this concept should be confirmed in further studies. To date, only one study (ARTDIVA trial in 30 patients) has been reported with ®ART Pure BRS device. At 6 months follow-up, TLF was 3.3% with no cardiac deaths or myocardial infarctions reported (Table 2) [27].
MAGMARIS
®Magmaris (Biotronik) BRS, renamed from ®DREAMS 2G, is a sirolimus-eluting BRS device with a resorbable magnesium alloy scaffold and a strut thickness of 150 µm. Metallic alloy backbone is thought to conform better lesion crossing, trackability, and pushability compared to polymeric scaffold BRS devices. The Magmaris program began with the AMS 1 stent (Biotronik AG, Bülach, Switzerland), which was bulky, hard to deliver, and limited by significant vessel recoil due to poor radial strength. This led to unacceptably high rates of TLR (45%) and major adverse cardiovascular events [MACE] (26.7%) [28]. Modifications of the strut design, the magnesium alloy, and the outer polymer matrix were incorporated into AMS 2 and AMS 3 stents, aimed at improving neointimal hyperplasia and vessel recoil. The AMS 3, the best-performing of these early BRS was renamed Drug Eluting AMS 1.0 (DREAMS) [29], leading to the first-in-man BIOSOLVE-I [30] clinical trial. The BIOSOLVE-I trial demonstrated significant improvements compared to the PROGRESS-AMS study, with TLR rates of 4.7% and TLF rates of 7% at 12 months, but still underperformed in comparison with contemporary stents [31]. With further improvements in design such as the incorporation of tantalum markers to enhance visualization, switching from a poly-D-lactc acid (PDLA) to a PLLA outer coating, and improved deployment technique, the ®DREAMS 2G scaffold was tested in the BIOSOLVE II and BIOSOLVE-III trials which enrolled stable patients with simple de novo lesions [32]. A recently presented pooled analysis of BIOSOLVE-II and BIOSOLVE-III demonstrated similar rates of TLF (6.4%, n=174) and clinically driven revascularization (3.7%, n=174) at 3 years follow-up when compared to DES without any event of scaffold thrombosis [33]. BIOSOLVE-IV confirms the safety and performance of the ®Magmaris scaffold in a large population with excellent device and procedure success and a very good safety profile up to 12 months in a low-risk population. This registry reported 3.9% TLR, 0.2% cardiac death, 1.1% target-vessel MI and 0.5% definite/probable scaffold thrombosis [34].
The scaffold is available in diameters of 3.0 and 3.5 mm and lengths of 15, 20, and 25 mm and is 6F guiding catheter compatible. Its resorption time is 12 months. Predilatation using a noncompliant balloon with a 1:1 balloon-to artery ratio is mandatory. The balloon should expand fully and the residual stenosis before ®Magmaris implantation should be <20%. Only one scaffold per lesion ought to be used. Postdilatation with a noncompliant (0.5 mm larger than the nominal scaffold size) is recommended at high pressure (>16 atm). In case of bailout situations, a second stent or scaffold could be used, preferably with proBIO coating to avoid electrochemical interaction [35]. A consensus paper by experts recommends against the use of ®Magmaris in situations such as STEMI, calcified lesions, poor medication compliance, or ostial lesions until further data is available. Its use should be restricted to stable patients with simple de novo lesions [36].
FANTOM
The ®Fantom stent (REVA Medical, San Diego, CA, USA), based on desaminotyrosine polycarbonate (DAT), has a 125-µm strut and incorporates iodine into the scaffold to improve visualization. Upon breakdown, the stent elutes sirolimus, with 80% of strut degradation occurring in the first 12 months and complete resorption occurring at 36 months. It conforms a comparable radial strength to that of contemporary metallic DES, low rates of recoil and easier device positioning facilitate by a radiopaque DAT polymer [37]. Initial results for FANTOM II study, Cohorts A and B have reported a TVF rate of 4.2% and definite device thrombosis rate of 0.4% at 12 months [37]. These results were maintained at 24 months [38] with TVF of 5.0% and definite or probable scaffold thrombosis of 0.8% (Table 2).
OTHER BIORESORBABLE SCAFFOLDS
MERES 100
The 100-µm-thick (Table 3) ®MeRes100 PLLA sirolimus-eluting scaffold (Meril Life Science) is a hybrid cell design (closed cells at the edges and open cells along the length) which is likely to degrade in 2–3 years. It allows optimal vessel wall conformability and high radial strength. It also has platinum radiopaque markers to improve device positioning. The scaffold has been preliminary tested in the first-in-man MeRes-1 study (n=108) that reported LLL 0.15±0.23 with no binary restenosis at 6 months. IVUS demonstrated a neointimal hyperplasia area of 0.14±0.16 mm2 while OCT showed a minimum scaffold area of 6.86±1.73 mm2 and 99.3% strut coverage. At 12 months, major adverse cardiac events occurred in only one patient (0.93%) with no scaffold thrombosis [39]. The extended outcomes of the MeRes-1 trial demonstrated sustained efficacy and safety of the ®MeRes100 BRS with maintained lumen patency up to two years by multimodality imaging and no very late scaffold thrombosis up to three-year clinical FU (Table 4) [40]. The MeRes-1 Extend study (a trail with 64 patients) [41] reported only 1.61% MACE rate at 2 years with just one case of ischemia-driven TLR and no case of scaffold thrombosis. In 24 patients who underwent OCT imaging, there was sustained flow from baseline and near complete strut coverage at 6-month follow-up. Late lumen loss at 6 months was 0.18 mm.
Table 3. Design characteristics of current bioresorbable scaffold technologies
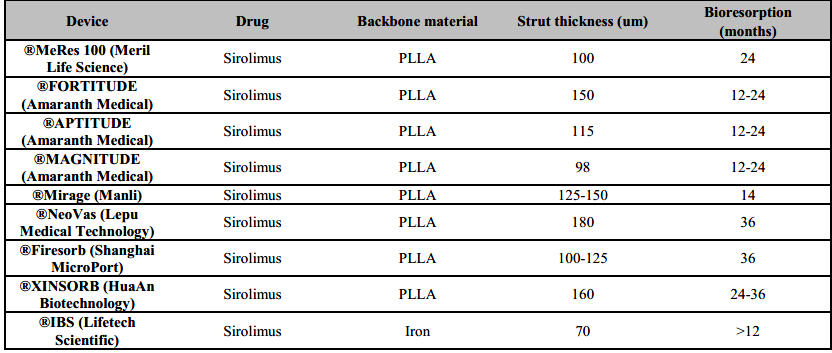
IBS: Iron bioresorbable scaffold; PLLA: Poly-L-lactic acid.
MIRAGE
The ®Mirage (Manli Cardiology) bioresorbable, microfibre, sirolimus-eluting scaffold is made from a PLLA-based scaffold with <5% PDLLA which has a helicoid structure, providing high flexibility. The struts are round with a thickness of 125 μm for scaffolds (Table 3) that are ≤3.0 mm in diameter and 150 μm for scaffolds that are ≥3.5 mm in diameter. Although the round shape of the scaffold might allow better embedment of the device, vessel area coverage is high at 40-47% [42]. When compared to ®Absorb BVS, ®Mirage BRS had comparable median in-scaffold LLL (0.37 mm versus 0.23 mm; P = 0.52) and TLF (17.2% versus 14.8%; P = 0.73) but higher angiographic median in-scaffold diameter stenosis (28.6% versus 18.2%; P = 0.046) and 3.4% definite subacute device thrombosis at 12 months (Table 4) [42].
MAGNITUDE
The Amaranth Medical polymer technology possesses special manufacturing properties displaying superior elongation at break compared with other PLLA-based BRS. A scaffold miniaturization process that preserves radial strength and over-expansion capabilities has been developed over time, spanning from the 150 µm (®FORTITUDE) to the 115 µm (®APTITUDE) and < 100 µm (®MAGNITUDE) iterations of the device (Table 3) [27]. In preliminary reports of the RENASCENT II study [43], which included 60 patients, 9-month follow-up with the ®APTITUDE device showed rates of TVF and target-vessel MI of 3.4% and 3.4%, respectively, and no occurrences of scaffold thrombosis. Furthermore, no additional TVFs or scaffold thrombosis occurred for up to 24 months. In preliminary reports of the RENASCENT III trial [44], which included 70 patients, 9-month follow-up with the ®MAGNITUDE device reported rates of TVF and binary restenosis of 6.3% and 7.9%, respectively without scaffold thrombosis was reported.
NEOVAS
The ®NeoVas (Lepu Medical Technology [Beijing]) is sirolimus-eluting BRS, which has a polymer backbone of PLLA (Table 3), with a strut thickness of 160 μm, coated with a 10µm layer of a 1:1 mixture of PDLLA and sirolimus (15.3µg/mm). The first-in-human study of the ®NeoVas BRS enrolled 31 patients and at 6 months showed a scaffold LLL of 0.26±0.32mm and one clinically driven TLR (3.2%), with no cardiac deaths or scaffold thrombosis [45]. Strut coverage measured with OCT was 95.7% at 6 months [45]. Subsequently, a randomized, controlled trial enrolled 560 patients, with 278 receiving the ®NeoVas BRS and 282 a CoCr-EES106. At 1 year, LLL was 0.14±0.36mm with the ®NeoVas BRS versus 0.11±0.34mm with the CoCr-EES [46]. OCT analysis showed higher strut coverage with the ®NeoVas NeoVas than with the CoCr-EES (98.7% versus 96.2%; P <0.001) and less strut malapposition (0.0% versus 0.6%; P <0.001). Of note, the two groups had similar clinical outcomes at 1 year [46].
XINSORB
The ®Xinsorb (HuaAn Biotechnology) sirolimus-eluting scaffold is made from PLLA, with a strut thickness of 160 μm, coated by PDLLA that releases 80% of the drug at 28 days. In a first- in-human trial on ®Xinsorb including 27 patients with a follow-up of 6 months, no MACE or scaffold thrombosis was observed, and in-scaffold angiographic LLL was 0.18 ± 0.21 mm [47]. In preliminary data at 30 months, clinically driven TLR was 6.7%, and scaffold thrombosis was 3.3% [48]. In a randomized, controlled study of 392 patients, 200 were randomly assigned to receive the ®Xinsorb and 192 to receive the ®Tivoli stents (Essen Technology (Beijing); a metallic sirolimus-eluting stent with a biodegradable polymer). At 12 months, there were similar rates of cardiac death, ischaemia-driven TLR and definite scaffold thrombosis in the two groups [49].
FIRESORB
The ®Firesorb scaffold (MicroPort) made from PLLA has a strut thickness of 100 μm for a 2.5 mm- diameter device and 125 μm for a 3.0 mm-diameter device, with a PDLLA abluminal coating that releases sirolimus (4µg/mm) [50]. The polymer resorption time is 36 months. In the first-in-human Future-I study on the ®Firesorb scaffold [50], which included 45 patients, one MI requiring revascularization had occurred at 2 years after implantation, but no definite or probable scaffold thrombosis or cardiac death occurred. 3-year clinical, angiography, IVUS, and OCT results of the FUTURE-I study demonstrated safety and feasibility of ®Firesorb BRS in the treatment of patients with single de novo coronary lesions [51]. The ongoing Future-II trial is likely to shed some more light.
IBS
The iron bioresorbable scaffold (®IBS; Lifetech Scientific) is a drug-eluting coronary scaffold with a strut thickness of 53 µm, with plasma nitride applied to a laser-cut, pure-iron scaffold to produce a nitride–iron scaffold that undergoes sequential coating by zinc electroplating (600nm) and then sirolimus (235µg/cm2) carrying amorphous PDLLA (12µm). The radial strength of the scaffold is equivalent to that of the CoCr-EES stent with the maximum expansion diameter of 4.4mm for a 3×18mm ®IBS scaffold [52]. Preclinical studies have been performed in rabbit and pig models, with 28-day results showing almost no corrosion and complete coverage of the scaffold, with minimal neointima with full luminal endothelialization. At 13 months after implantation in the rabbit model, scaffold struts could not be identified with the use of OCT, and the struts appeared dim and small with ongoing corrosion when imaged with micro-CT [53]. A first-in-man study of IBS ([IBS-FIM], (NCT03509142) is evaluating it is the feasibility, preliminary safety and efficacy.
FUTURE DIRECTIONS
SCAFFOLD DESIGN CONSIDERATION
Strut thickness is one of the principal mechanisms behind the rates of stent thrombosis seen with early-generation BRS [54]. Thicker struts (greater than 150 µm) are required to provide enough radial strength to prevent vessel recoil. However, it leads to longer resorption times. The polymer dismantling and scaffold discontinuity, have also been associated with thicker strut designs leading to adverse events [55]. The latest generations of BRS ([®MeRes100, Meril Life Science, Gujarat, India]; [®Mirage, Manli Cardiology, Singapore]; [®MAGNITUDE, Amaranth Medical, Mountain View, CA, USA]; [®Firesorb, MicroPort, Shanghai, China]) through innovations in stent design have reduced strut thickness [56]. Thinner struts have shown to improve deliverability, reduce the shear stress at the vessel wall, allowing for less turbulent blood flow, improved endothelization, and reduced thrombus formation. Thinner struts have also been shown to reduce restenosis and periprocedural MI rates. Through these innovations in stent and strut design, lower-profile scaffolds have been made possible without sacrificing radial strength. Trials are underway to evaluate whether these innovations are going to have improved outcomes compared to early-generation devices [55].
PROCEDURAL CONSIDERATION
The importance of correct deployment technique cannot be underestimated despite improvements in scaffold design. The adverse outcomes in early clinical trials were attributed to two common procedure related factors such as underexpansion and malapposition [57]. By mitigating the scaffold underexpansion and malapposition the incidence of stent thrombosis was reduced significantly in early-generation BRS reduced [58, 59]. The 4P technique (patient selection, proper sizing, pre-dilation, and post-dilation) is similar and is aimed at preventing under expansion and malposition when using current BRS. Of note, future trials should only proceed with such mandatory procedural steps to mitigate these risks and ensure a favourable result [56].
PATIENT AND LESION CONSIDERATION
Vessel size is a key factor, with small vessels (<2.25 mm) displaying higher rates of scaffold thrombosis and stent thrombosis [60], and large vessels (>3.75 mm) risking underexpansion or scaffold fracture [61]. Other complex anatomical subsets such as ostial lesions, bifurcation, severely calcified lesions [62], and in-stent restenosis [63] have all shown inferior outcomes in clinical trials. Patient-related factors and the clinical presentations of patients also play a role. When used in STEMI, both magnesium- and lactate-based scaffolds have demonstrated inferior event rates compared with everolimus DES [64, 65]. When used in non-STEMI patients, the ®Magmaris appears to be safe when compared to EES at 12 months, but long-term data are warranted [66]. Future clinical trials should aim to adequately assess the safety and efficacy of BRS in higher-risk anatomic and patient subsets. With improvements in scaffold design, preservation of vessel lumen and vasomotion may become possible in situations such as in-stent restenosis and chronic total occlusions.
DUAL ANTIPLATELET THERAPY CONSIDERATION
The consideration of dual antiplatelet therapy (DAPT) duration requires understanding of the BRS resorption time. During resorption, scaffold discontinuity and polymer breakdown may provide a nidus of thrombus formation, so DAPT should be maintained until complete scaffold resorption is achieved. As with all DAPT, however, this needs to be balanced against the increased risk of bleeding events. Technology advances of BRS and quicker resorption times are likely to shorten DAPT duration. Current European guidelines treat polymeric and metallic resorbable scaffolds as a single class and recommend a minimum of 12 months of DAPT (class IIA C) [67].
CONCLUSIONS
The best clinical data from the Absorb shows that outcomes with first generation BRS are inferior to contemporary DES and should not be used in clinical practice. The setback this experience has diminished the exuberant enthusiasm, but not the rationale for their continued modifications for BRS. It is expected that next generations of BRS undergo iterations to improve physical characteristics for better acute and long-term outcome. At present, the use of BRS is reserved for simple de novo lesions. However, rigorous adequately powered with long follow-up trials are warranted to establish the role of current BRS in the treatment of complex lesions.