My SciELO
Services on Demand
Journal
Article
Indicators
-
Cited by SciELO
-
Access statistics
Related links
-
Cited by Google
-
Similars in SciELO
-
Similars in Google
Share
Revista de Osteoporosis y Metabolismo Mineral
On-line version ISSN 2173-2345Print version ISSN 1889-836X
Rev Osteoporos Metab Miner vol.12 n.4 Madrid Oct./Dec. 2020 Epub Apr 05, 2021
https://dx.doi.org/10.4321/s1889-836x2020000400007
REVIEW
Osteocalcin: from marker of bone formation to hormone; and bone, an endocrine organ
1Hospital Support Team. Palliative care. University Hospital of Móstoles. Móstoles. Madrid (Spain)
2Department of Traumatology and Orthopedic Surgery. Jiménez Díaz Foundation Health Research Institute. Jiménez Díaz Foundation. Madrid (Spain)
Osteocalcin is a protein synthesized by the osteoblast. Before being released into the extracellular matrix, human osteocalcin undergoes gamma-carboxylation, as gamma-carboxy-glutamic acid binds at positions 17, 21 and 24. Part of the carboxylated and decarboxylated osteocalcin passes into the circulation. Since its discovery in the late 70s, it has been used as a marker of bone formation as it is an osteoblastic product and its role in the body is unknown. In recent years, osteocalcin has been identified as a hormone. Bone is considered an endocrine organ. Osteocalcin acting as a hormone is the decarboxylated form. Osteocalcin is involved in glucose homeostasis, skeletal muscle function, brain development, male fertility, hepatic steatosis, and arterial calcification. All of these facts have actually been tested in mice, but there is strong evidence that this could occur in humans. We are faced with facts that, if proven, would have enormous clinical significance.
Key words osteocalcin; hormone; glucose; insulin; skeletal muscle; brain development; hepatic steatosis; arterial calcification
Osteocalcin is a protein synthesized by the osteoblast. It was identified in the late 1970s and in humans contains 49 amino acids1. Before being released into the extracellular matrix, osteocalcin undergoes gamma-carboxylation, as gamma-carboxy-glutamic acid binds at positions 17, 21 and 24. A gamma-carboxylase is involved in this reaction and the presence of vitamin K is required (Figure 1). The presence of the two carboxyl groups causes gamma-carboxylated osteocalcin to have a high affinity for calcium and, when released into the extracellular environment, binds in a large proportion to hydroxyapatite in bone. A part of this gamma-carboxylated osteocalcin and also non-carboxylated osteocalcin remain in the circulation2. Only 10-30% of the synthesized osteocalcin reaches the circulation, and the rest remains attached to the bone matrix. Non-carboxylated osteocalcin represents 1/3 of total osteocalcin. During resorption, when the bone matrix is destroyed, part of the osteocalcin that is bound to the bone passes into the circulation2. Osteocalcin is only synthesized by osteoblasts and is the most abundant non-collagenous protein in the extracellular matrix and is the tenth most abundant protein in vertebrates3. Since first reported, its levels were correlated with bone formation4. For all researchers working in bone metabolism, having a new bone formation marker was a breakthrough when the only markers of remodeling that were available up to that time were hydroxyproline and total alkaline phosphatase. The bone isoenzyme of alkaline phosphatase could also be measured by a rather complex method by electrophoresis. Osteocalcin has been used for many years as a marker of bone formation in practically all the work carried out in this regard. It is used less since 2011 when the International Osteoporosis Foundation (IOF) and the International Federation of Clinical Chemistry and Laboratory Medicine (IFCC) recommended that the N-terminal propeptide of type I collagen (PINP) be used as a marker of formation and the C-terminal β-telopeptide of type I collagen or β-crosslaps (β-CTX) as a marker of resorption in clinical studies on osteoporosis5.
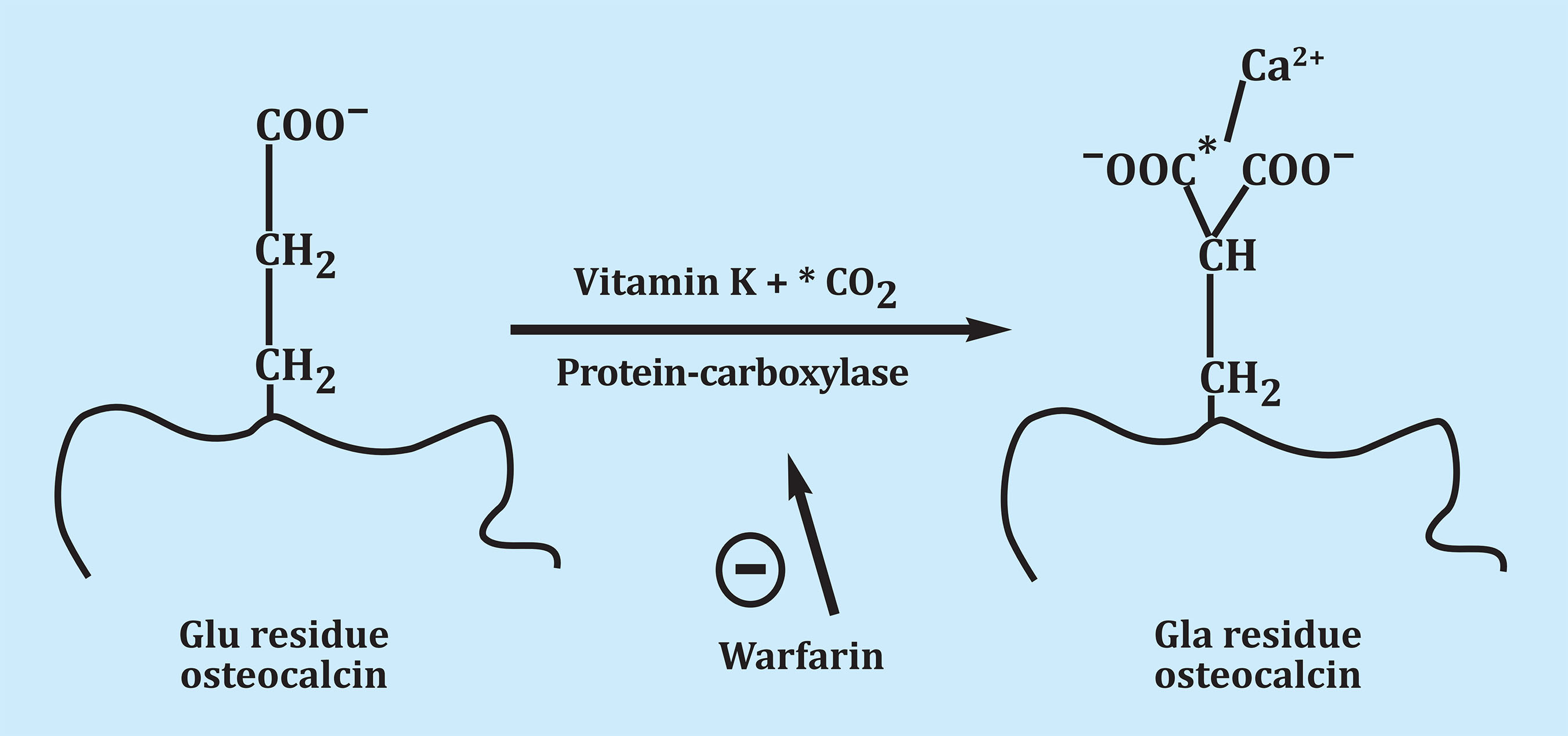
Figure 1. By post-translational carboxylation, the glutamic acid of osteocalcin is transformed into gamma-carboxy glutamic acid. The presence of the two carboxyl groups causes a high affinity for the calcium Ca2+ binding of the extracellular matrix
For many years, and despite its abundance, the role of osteocalcin in the body was unknown. Due to its post translational modification, it was thought to be involved in bone mineralization. But when mice without osteocalcin (osteocalcin-/-) were obtained, it was found that these mutants presented totally normal mineralization, making it clear that the function of osteocalcin was not related to bone mineralization6.
Bone contains osteoclasts, cells whose function is to destroy bone, and this active destruction of mineralized bone requires energy7. Bone formation also requires energy8. Thus, researcher groups such as Karsenty et al. hypothesized that bone modeling and remodeling must be associated with the regulation of energy metabolism9,10. Probably, the amount of energy from the active destruction of the bone is proportional to the surface occupied by it. This energy requirement is probably very high, since bone resorption does not occur as an isolated event, but in the context that there must be a coordinated regulation of a biphasic function called modeling during childhood and remodeling in adult life. This is why the aforementioned authors hypothesized that bone modeling and remodeling must be linked to the regulation of energy metabolism. This vision of a coordinated regulation of bone mass and energy metabolism is supported by clinical evidence. For example, longitudinal bone growth stops in children and bone mass declines in adults with severely limited access to food (ie, energy)11. Furthermore, another link between bone remodeling and energy metabolism is that bone mass always declines in both sexes when gonadal function decreases. Considering these observations, it is concluded that there must be a coordinated regulation of bone growth/mass, energy metabolism and reproduction9.
We previously mentioned that mice (osteocalcin-/-) had normally mineralized bones, but they develop some phenotypes that can only be explained, given the site of synthesis of osteocalcin and its abundance, if this molecule were acting as a hormone. Indeed, the mutant mice (osteocalcin-/-) had more visceral fat than the controls and also had fewer offspring. The systematic study of these phenotypes established that bone must be an endocrine organ and that the hormone it secretes, osteocalcin, affects energy metabolism and fertility. In other words, there is a coordinated regulation, endocrine in nature of energy metabolism and reproduction. A fundamental part is that the bone would be an endocrine organ and not the receptor of the action of hormones.
At this time, the knowledge of the mechanisms of action of osteocalcin in its target organs is a work in progress. For example, increased adiposity in mice (osteocalcin-/-) could be associated with a decrease in energy expenditure and not an increase in appetite (another function regulated by bone). The molecular basis of this phenomenon has not yet been discovered10.
OSTEOCALCIN AND GLUCOSE HOMEOSTASIS
In a study by Wei et al.9, in which they cultured mouse osteoblasts with pancreatic islets, increased insulin expression in the islets was observed. Osteoblasts did not increase the expression of any other hormones secreted by the pancreatic islets. When osteoblasts from mice (osteocalcin-/-) were added, this insulin expression did not occur. Thus, it was shown that osteoblasts are endocrine cells that regulate insulin expression and that osteocalcin is the hormone responsible for this action. Mice (osteocalcin-/-) on a normal diet were also found to be hyperglycemic and hypoinsulinemic. Insulin secretion was decreased in the absence of osteocalcin.
In this same study, a glucose tolerance test showed that the mice (osteocalcin-/-) were glucose intolerant because they had a decrease in insulin expression. Wei et al.9 stated that the fact that osteocalcin regulates glucose metabolism is not synonymous with bone being the origin of diabetes. It simply increases the regulatory landscape of glucose metabolism.
Research carried out in rats with normal diet shows that osteocalcin is necessary and sufficient to promote the proliferation of β cells in the pancreatic islets, to promote the expression and secretion of insulin and to promote glucose uptake in peripheral tissues. and hence glucose homeostasis12.
The receptor that mediates the osteocalcin signal in β-pancreatic cells and other peripheral tissues is a G-protein coupled receptor called GPR6a13. Specific gene deletion and other genetic experiments have established that, in vivo, osteocalcin is, without a doubt, the ligand that explains the regulation of glucose homeostasis through GPR6a10. The biological importance of the regulation of osteocalcin in glucose homeostasis has been verified in normal mice fed a high fat diet. Exogenous osteocalcin almost completely rescues glucose intolerance in these animals14.
It was very important to know if these actions were produced by carboxylated or decarboxylated osteocalcin. In bacteria there is no gamma-carboxylation and thus recombinant bacterial osteocalcin that was not gamma-carboxylated could be produced. This non-carboxylated osteocalcin was able to induce insulin expression in the pancreatic islets, indicating that the non-carboxylated form of osteocalcin is the one that acts as a hormone9.
Osteoblasts have insulin receptors on their surface, and it is of great interest that insulin and osteocalcin are involved in a regulatory loop. So the insulin signal in the osteoblasts is required for good glucose homeostasis throughout the body9.
Mice that do not have insulin receptors on osteoblasts, when eating a normal diet, experience a decrease in the active circulating form of osteocalcin, a decrease in insulin secretion, glucose intolerance and insulin resistance. The insulin signal on osteoblasts inhibits the expression of osteoprotegerin and favors bone resorption. The low pH that occurs under osteoclasts favors the formation of decarboxylated osteocalcin, which is the active form of osteocalcin. This active osteocalcin acts again on the β-pancreatic cells and new insulin is formed that acts again on the osteoblast and the cycle begins again (Figure 2)15.
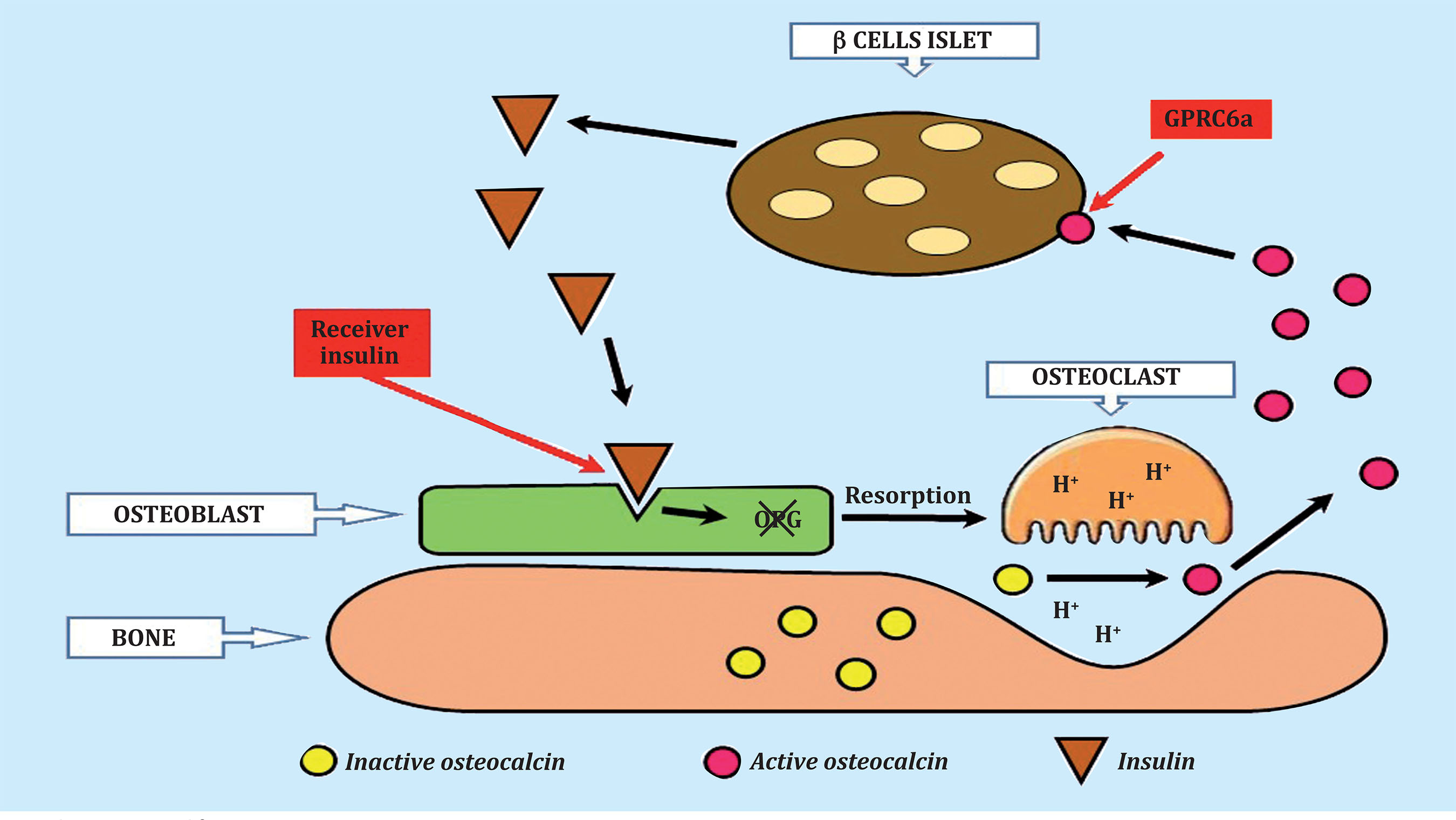
Figure 2. Osteocalcin-insulin cycle. Decarboxylated osteocalcin, the active form, binds to the GPRC6a receptor on pancreatic ß cells, causing the release of insulin. Insulin then binds to the insulin receptor on the osteoblast, causing a decrease in osteoprotegerin synthesis and an increase in bone resorption. In the acidic environment of the osteoclast, the inactive, gamma-carboxylated osteocalcin becomes active, non-carboxylated. Active osteocalcin restarts the cycle by binding to the ß cells of the pancreatic islets
OSTEOCALCIN AND SKELETAL MUSCLE
A simple injection of exogenous osteocalcin immediately before exercise or chronic administration of this hormone for 1 month, not only increases the exercise capacity of young mice but also restores the exercise capacity of older mice16. At the same time as increasing muscle strength in aged mice, chronic administration of osteocalcin promotes muscle mass gains17. That is, exogenous osteocalcin is not only necessary but sufficient to reverse the decline in exercise capacity and muscle mass observed in older mice18.
Osteocalcin signal on skeletal muscle is carried out through the GPR6a receptor18. Osteocalcin has been shown to regulate nutrient uptake and catabolism in muscle during exercise. Glucose, the main nutrient used by skeletal muscle to generate energy during exercise, is stored in myofibers as glycogen. The degradation of glycogen in skeletal muscle during exercise is lower in mice that do not have the GPR6a receptor and in mice (osteocalcin-/-), showing that osteocalcin favors glycogenolysis.
It has also been observed that the accumulation of tricarboxylic cycle intermediates in skeletal muscle seen in mice after exercise is not observed in mice (GPR6a-/-), indicating that there is no ATP input from the tricarboxylic cycle. Over an extended period of exercise, when animals deplete their glycogen stores, the uptake and catabolism of fatty acids increases in skeletal muscle19. Osteocalcin favors the oxidation of fatty acids in myofibers. The osteocalcin signal in the myofibers promotes the uptake and catabolism of glucose and fatty acids during exercise, which is why a decrease in physical activity is observed in mice (osteocalcin-/-) and (GPR6a-/-) when compared to controls20.
For decades it has been common knowledge that exercise induces changes in the immune system21. Interleukin 6 (IL6) was the first molecule that was seen to be released into the blood in response to muscle contraction22. IL6 promotes glucose uptake and fatty acid oxidation in skeletal muscle, increasing glucose production in the liver and lipolysis in adipose tissue23.
Exercise induces bone resorption and the production of bioactive osteocalcin. Mice (IL6-/-) after exercise do not increase bone resorption markers or bioactive osteocalcin, as happens in healthy mice. This suggests the existence of a loop between bone (via osteocalcin) and muscle (via IL6) that promotes adaptation to exercise20. Thus:
IL6 and osteocalcin regulate similar aspects of skeletal metabolism during exercise, increasing glucose and fatty acid catabolism.
But, does osteocalcin act independently of IL6 in these processes? Mera et al.16 studied the action of osteocalcin on a myofiber culture in normal mice and mice (IL6-/-). Osteocalcin induced these functions in the two types of culture, which reveals that IL6 is not required for the catabolic action of osteocalcin in skeletal muscle.
The identification of a bridge between bone, via osteocalcin, and skeletal muscle, via IL6, which is necessary and sufficient to promote adaptation to exercise in young and old animals, represents a significant advance in our understanding of how the skeletal muscular system controls a function that is essential for the survival of all vertebrates20.
OSTEOCALCIN AND BRAIN DEVELOPMENT
Osteocalcin is necessary for the development of the brain and for its function. Its absence in mice produces a profound deficit in spatial knowledge and memory and an exacerbation of anxiety. Ostecalcin could prevent lowering of consciousness due to age25.
The brain-derived neurotrophic factor BDNF, a wellknown molecule that participates in memory, dependent on the hippocampus, is the mediator of the regulation of osteocalcin on cognitive function26. Non-carboxylated osteocalcin is able to stimulate the dynamics of BDNF vesicle transport towards sypnasis in rat neurons25.
Khrimian et al.27 identified the receptor that transfers the signal from osteocalcin to neurons. It is Gpr158, a G protein-bound receptor expressed on neurons in the CA3 region of the hippocampus, which transmits the osteocalcin signal through inositol 1,4,5-triphosphate and a brain-derived neurotrophic factor. This is very important for future therapeutic actions.
OSTEOCALCIN AND MALE FERTILITY
Decarboxylated osteocalcin acts on the Leydig cells of the testes, promoting the biosynthesis of testosterone28. Decarboxylated osteocalcin acts through the GPRC6a receptor, as it did on β-pancreatic cells.
Osteocalcin has also been shown to act via a pancreas-bone-testes axis that regulates, independently and in parallel to the hypothalamic-pituitary-testes axis, male reproductive function, promoting testosterone biosynthesis29.
In mice on a high-fat diet, daily injections of osteocalcin of 3 or 30 ng/g of body weight/day partially restore insulin sensitivity and glucose tolerance. Furthermore, mice treated with intermittent injections of osteocalcin exhibited increased energy expenditure and were protected from diet-induced obesity. Finally, the fatty diet-induced hepatic steatosis was completely avoided in the mice that received osteocalcin daily. These results show that daily ostecalcin injections improve glucose management and prevent the development of type 2 diabetes14.
OSTEOCALCIN AND ARTERIAL CALCIFICATION
In the "bone-vascular calcification paradox" there is high calcification in the vessels, leading to arterial stiffness and cardio-vascular disease, and reduced calcification in the bone leading to osteoporosis and bone fracture. This leads to the hypothesis that bone metabolism and cardiovascular disorders could have common pathogenic pathways, leading to the expression “bone-vascular axis”30. Several molecules seem to play a role in this axis, and one of them would be osteocalcin.
Rashdan et al. maintain the hypothesis that osteocalcin regulates the calcification of vascular smooth muscle cells31. Immunohistochemistry reveals the co-localization of osteocalcin with calcification of vascular smooth muscle cells in calcified plaques of carotid arteries. Osteocalcin involvement in the development of arteriosclerosis is supported by a recent meta-analysis by Millar et al., in which a relationship between osteocalcin and atherosclerosis markers is observed in histological samples32. In this same study, the authors found no differences between osteocalcin levels in patients with and without vascular events. That is, osteocalcin seems to be a marker only of the calcification process.
OSTEOCALCIN IN HUMANS
So far, all the review carried out on the role of osteocalcin as a hormone has focused on experiments in mice or rats. It is extremely important to know if these facts can be transferred to humans, with the clinical implications that this would entail.
A systematic review of the literature conducted between 2007 and 2014 identified 82 studies that observed that serum levels of decarboxylated or total osteocalcin are negatively correlated with blood glucose, insulin resistance, obesity, or markers of metabolic syndrome. Furthermore, some of the human data support a role for osteocalcin in insulin secretion33.
Treatment with bisphosphonates has been found to decrease non-carboxylated osteocalcin in serum and that levels of it and/or markers of insulin sensitivity or secretion are positively correlated with markers of bone resorption in humans34.
It has also been seen that patients with a dominant form of osteopetrosis due to a defect in osteoclast activity are characterized by decreased levels of decarboxylated osteocalcin and hypoinsulinemia15.
Changes in osteocalcin levels, following bisphosphonate treatments, are associated with changes in body mass and fat35.
Oury et al. analyzed a cohort of patients with testicular failure and identified 2 individuals with a variant in one of the GPRC6a domains. These patients had glucose intolerance and insulin resistance36.
Osteocalcin levels have been compared between patients with type 2 diabetes mellitus and the non-diabetic population, with diabetics having lower levels of osteocalcin37. Patients with metabolic syndrome also have lower levels of total osteocalcin than healthy individuals.
In addition, there is a correlation between total and decarboxylated osteocalcin with markers of glycemic status and other cardio-metabolic parameters38. These authors point out the need to delve into these findings and their possible participation in human health, as well as analyze their possible therapeutic potential.
An observational study assessed the association between serum levels of osteocalcin and knowledge capacity in healthy adults, showing that it is positively correlated with measures of global knowledge in elderly women39. In children and adolescents with nonalcoholic fatty liver, the concentration of osteocalcin is inversely correlated with liver enzymes and with the severity of the disease40.
Smith et al., in a study carried out in 202041, considered the normal values of decarboxylated and carboxylated osteocalcin in adult men. These values should be included in future studies in clinical trials and associated with the prediction of events such as fractures or risk of diabetes. The amount of evidence on the multi-organ effects of decarboxylated osteocalcin, supported by the facts demonstrated in vivo and in vitro, indicates the need to deepen these findings and its possible participation in human health, as well as to analyze its possible therapeutic potential.
CONCLUSIONS
The discoveries made in recent years concerning the role of osteocalcin as a hormone and bone as an endocrine organ are truly surprising. Osteocalcin, which for bone metabolism researchers was simply a marker of bone formation with no known function.
In mice, osteocalcin is involved in glucose homeostasis, skeletal muscle function, brain development, hepatic steatosis, male fertility, and arterial calcification.
We begin to find works that seem to anticipate that this could also happen in humans.
It is extremely important to address this type of research in humans, because if what happened in humans were similar to what happens with osteocalcin in mice, the therapeutic implications of this compound would be extremely interesting.
Bibliografía
1 Price PA, Otsuka AA, Poser JW, Kristaponis J, Raman N. Characterization of a gamma-carboxyglutamic acid-containing protein from bone. Proc Natl Acad Sci USA. 1976;73:1447-51. [ Links ]
2 Poser JW, Esch FS, Ling NC, Price PA. Isolation and sequence of the vitamin K-dependent protein from human bone. Undercarboxylation of the first glutamic acid residue. J Biol Chem. 1980;225:8685-91. [ Links ]
3 Hauschka PV, Lian JB, Cole DE, Gundberg CM. Osteocalcin and matrix Gla protein: vitamin K dependent proteins in bone. Physiol Rev. 1989;69:990-1047. [ Links ]
4 Delmas PD, Eastell R, Garnero P, Seibel MJ, Stepan J. The use of biochemical markers of bone turnover in osteoporosis. Committee of Scientific Advisors of the International Osteoporosis Foundation. Osteoporosis Int. 2000;11 (Supl 6):S2-17. [ Links ]
5 Vasikaran S, Eastell R, Bruyère O, Foldes AJ, Garnero P, Griesmacher A, et al. Markers of bone turnover for the prediction of fracture risk and monitoring of osteoporosis treatment: a need for international reference standards. Osteoporosis Int. 2011;22:391-420. [ Links ]
6 Ducy P, Desbois C, Boyce B, Pinero G, Stacy B, Dunstan C, et al. Increased bone formation in osteocalcin-deficient mice. Nature. 1996;382:448-52. [ Links ]
7 Kim JM, Jeong D, Kang HK, Jung SY,Lee SH, Choi Y, et al. Osteoclast precursors display dinamic metabolic shifts toward accelerated glucose metabolism at an early stage of RANKL-stimulated osteoclast differentiation. Cell Physiol Biochem. 2007;20:935-46. [ Links ]
8 Karsenty G, Kronenberg HM, Settembre C. Genetic control of bone formation. Annu Rev Cell Dev Biol. 2009;25:629-48. [ Links ]
9 Wei J, Karsenty G. An overview of the metabolic function of osteocalcin. Rev Endocr Metab Disord. 2015;16:93-8. [ Links ]
10 Karsenty G. Update on the biology of osteocalcin. Endocr Pract. 2017;23:1270-4. [ Links ]
11 Misra M, Klibansky A. The neuroendocrine basis of anorexia nervosa and its impact on bone metabolism. Neuroendocrinology. 2011;93:65-73. [ Links ]
12 Lee NK, Sowa H, Hinol E, Ferron M, Ahn JD, Confavreux C, et al. Endocrine regulation of energy metabolism by the skeleton. Cell. 2007;130:456-69. [ Links ]
13 Wei J, Hanna T, Suda N, Karsenty G, Ducy P. Osteocalcin promotes b-cell proliferation during development and adulthood through Gprc6a. Diabetes. 2014;63:1021-31. [ Links ]
14 Ferron M, McKee MD, Levine RL, Ducy P, Karsenty G. Intermittent injections of osteocalcin improve glucose metabolism and prevent type 2 diabetes in mice. Bone. 2012;50:568-75. [ Links ]
15 Ferron M, Wei J, Yoshizawa T, Del Fattore A, DePinho RA, Teti A, et al. Insulin signaling in osteoblasts integrates bone remodeling and energy metabolism. Cell. 2010;142:296-308. [ Links ]
16 Mera P, Laue K, Ferron M Confavreux C, Wei J, Galan-Diez M, et al. Osteocalcin signaling in myofibers is necessary and sufficient for optimum adaptation to exercise. Cell Metab. 2016;23:1078-92. [ Links ]
17 Mera P, Laue K, Wei J, Berger JM, Karsenty G. Osteocalcin is necessary and sufficient to maintain muscle mass in older mice. Mol Metab. 2016;5:1042-7. [ Links ]
18 Pedersen BK, Steensberg A, Fischer C, Keller C, Keller P, Plomgaard P, et al. Searching for the exercise factor: is IL6 a candidate?. Muscle Res Cell Motil. 2003;24:113-9. [ Links ]
19 Hawley JA, Hargreaves M, Joyner MJ, Zierath JR. Integrative biology of exercise. Cell. 2014;159:738-49. [ Links ]
20 Karsenty G, Mera P. Molecular bases of the crosstalk between bone and muscle. Bone. 2018;115:43-9. [ Links ]
21 Pedersen BK, Hoffman-Goetz L. Exercise and the immune system: regulation, integration and adaptation. Physiol Rev. 2000;80;1055-81. [ Links ]
22 Overmaier KA, Evans CR, Qi NR, Minogue CE, Carson JJ, Chermside-Scabbo CJ, et al. Maximal oxidative capacity during exercise is associate with skeletal muscle fuel selection and dynamic changes in mitochondrial acetylation. Cell Metab. 2015;21:468-78. [ Links ]
23 Febbraio MA, Hiscock N, Sachetti M, Fischer CP, Pedersen BK. Interleukin 6 is a novel factor mediating glucose homeostasis during skeletal muscle contraction. Diabetes. 2004;53:1643-8. [ Links ]
24 Van Hall G, Steensberg A, Sachetti M, Fisher C, Keller C, Schjerling P, et al. Interleukin 6 stimulates lipolysis and fat oxidation in humans. J Clin Endocrinol Metab. 2003;88:3005-10. [ Links ]
25 Obri A, Khrimian L, Karsenty G, Oury F. Osteocalcin in the brain: from embryoncontextualic development to agerelated decline in cognition. Nat Rev Endocrinol. 2018;14:174-82. [ Links ]
26 Hall J, Thomas KL, Everitt BJ. Rapid and selective induction of BDNF expression in the hippocampus during contextual learning. Nat Neurosci. 2000;3:533-5. [ Links ]
27 Khrimian L, Obri A, Ramos-Brossier, Rousseaud A, Moriceau S, Nicot AS, et al. Gpr158 mediates osteocalcin´s regulation of cognition. J Exp Med. 2017; 214:2859-73. [ Links ]
28 Oury F, Sumara G, Sumara O, Ferron M, Chang H, Smith CE, et al. Endocrine regulation of male fertility by the skeleton. Cell. 2011;144:796-809. [ Links ]
29 Karsenty G, Oury F. Regulation of male fertility by the bone-derived hormone osteocalcin. Mol Cell Endocrinol. 2014; 382:1-13. [ Links ]
30 D´Onofrio L, Maddaloni E, Buzzeti R. Osteocalcin and sclerostin: background characters or main actors in cardiovascular disease? Diabetes Metab Res Rev. 2020;36:e3217. [ Links ]
31 Rashdan NA, Sim AM, Lin C, Phadwal K, Roberts F, Carter R, et al. Osteocalcin regulates arterial calcification via altered Wnt signalling and glucose metabolism. J Bone Miner Res. 2020;35:357-67. [ Links ]
32 Millar SA, Patel H, Anderson SI, England TJ, O´Sullivan SE. Osteocalcin, vascular calcification and arterosclerosis: a systematic review and metaanalysis. Front Endocrinol (Lausanne). 2017;8:183. [ Links ]
33 Ferron M, Lacombe J. Regulation of energy metabolism by the skeleton: osteocalcin and beyond. Arch Biochem Biophys. 2014;561:137-46. [ Links ]
34 Thrailkill KM, Jo CH, Cockrell GE, Moreau CS, Lumpkin CK Jr, Fowlkes JL. Determinants of undercarboxylated and carboxylated osteocalcin in type 1 diabetes. Osteoporosis Int. 2012;23:1799-806. [ Links ]
35 Schafer AL, Sellmeyer DE, Schwartz AV, Rosen CJ, Vittinghoff L, Palermo L, et al. Change in undercarboxylated osteocalcin is associated with changes in body weight, fat mass, and adiponectin: parathyroid hormone (1-84) or alendronate therapy in postmenopausal women with osteoporosis (the PaTH study). J Clin Endocrinol Metab. 2011;96:E1982-9. [ Links ]
36 Oury F, Ferron M, Huizen W, Confavreux C, Wei J, Galan-Diez M, et al. Osteocalcin regulates murine and human fertility through a pancreas-bone-testis axis. J Clin Invest. 2013;123:2421-33. [ Links ]
37 Liu C, Wo J, Zhao Q, Wang Y, Wang B, Zhao Wl. Association between serum total osteocalcin level and type 2 diabetes mellitus: a sistematic review and meta-analysis. Horm Metab Res. 2015;47:813-9. [ Links ]
38 Luo Y, Ma X, Hao Y, Xiong Q, Xu Y, Pan X, et al. Relationship between serum osteocalcin level and carotid intimamedia thickness. Horm Metab Res 2015;47:813-819. Cardiovascular Diabetol. 2015;14:82. [ Links ]
39 Bradburn S, McPhee JS Bagley L, Sipila S, Stenroth L, Narici MV, et al. Association between osteocalcin and cognitive performance in healthy older adults. Age Ageing. 2016;45:844-9. [ Links ]
40 Amin S, El Amrousy D, Elrifaey S, Gamal R, Hodeib H. Serum children osteocalcin levels with nonalcoholic fatty liver disease. J Pediatric Gastroenterol Nutr. 2018;66:117-21. [ Links ]
41 Smith C, Voisin S, Al Saedi A, Phu S, Brennan-Speranza T, Parker L, et al. Osteocalcin and its forms across the lifespan in adult men. Bone. 2020;130: 115085. [ Links ]
Received: November 30, 2020; Accepted: January 09, 2021