Highlights
Several studies have demonstrated the therapeutic potential of naringin against diabetes, allergies, asthma, osteoporosis, hypertension and cancer, as well as protection of gastrointestinal tract. However, the antineoplastic effects of naringin in bladder cancer have been poorly studied. Our results showed naringin presented cytotoxic effects, reduced the number of colonies and inhibited cell migration, demonstrating naringin could be a promising candidate for bladder cancer treatment.
Introduction
Worldwide, 0.573 million new cases and almost 0.212 million deaths due to bladder cancer were estimated in 20201. Urothelial carcinoma and transitional cell carcinoma (TCC) are the most common type and subtype of bladder cancer, respectively. About 75 % of urothelial carcinoma patients have tumors confined to the bladder mucosa or submucosa, which are usually treated with transurethral resection of the tumor and intravesical therapy2. On the other hand, for that 25 % of patients with muscle-invasive disease, more aggressive therapy is necessary, including partial or total cystectomy and systemic chemotherapy with methotrexate, vinblastine, doxorubicin, and cisplatin, protocol known as MVAC3.
The main difficulty found for cancer therapy is the toxicity accompanied by high failure rates, which justifies the search for potential natural products as a therapeutic alternative4. Because of its diverse chemical structure, bioactive natural products are capable of modulating the cancer microenvironment and diverse cell signaling pathways5.
Among natural compounds with pharmacological potential, naringin, a flavonoid found mainly in citrus fruits, has been gaining attention because of its anti-inflammatory, antioxidant, and antitumor properties6. There is strong evidence for the association between treatment with naringin and antitumor activity, through modulation of several signaling cascades involved in cell proliferation, autophagy, apoptosis, angiogenesis, metastasis, and invasion7. Despite data showing that naringin can induce antiproliferative effects in many types of cancer cells, there is only one study demonstrating the effects of naringin on bladder cancer cells8.
In this context, this study aimed to investigate the antineoplastic potential of the naringin in silico and in vitro on bladder cancer cells by exploring its effect on cell proliferation, morphology, migration, cell cycle progression, and its ability to induce mutagenic effects.
Methods
Quantitative structure-activity relationship (QSAR) analysis
The naringin structure (Figure 1) was subjected to quantitative structure-activity relationship (QSAR) analysis using the platform Prediction of Activity Spectra for Substances (PASS online) to predict its antitumor potential and possible mechanisms of action. The naringin is a disaccharide derivative that is (S)-naringenin substituted by a 2-O-(α-L-rhamnopyranosyl)-β-D-glucopyranosyl moiety at position 7 via a glycosidic linkage (PubChem CID 442428). The results are provided as a probability that the compound is active (Pa) and inactive (Pi). Fourteen antitumor effects and mechanisms of action related to the tumorigenesis process were analysed. The results were expressed by difference Pa-Pi and were classified as Pa-Pi < 0.2: low potential, Pa-Pi ≥ 0.5: moderate potential and Pa-Pi ≥ 0.5: high potential9.
Cell lines and naringin
The cell lines 5637 and T24 from urinary bladder tumors were kindly donated by Dr. Daisy Maria Fávero Salvadori from Universidade do Estado de São Paulo (UNESP), Brazil. The cell lines were maintained as described by Da Silva et al10. Naringin (≥ 95%) was purchased from Sigma-Aldrich (Saint Louis, EUA) and was solubilized at 400 µM in culture medium with 2 % dimethylsulfoxide (DMSO) immediately before its use. Previous study of our research group has demonstrated that 2% DMSO does not alter the viability of 5637 and T24 cells11.
Cytotoxicity
Cytotoxicity was assessed using the Cell Proliferation Kit II (XTT), from Roche Diagnostics (Manheim, Germany). Briefly, 1.0 x 104 cells/well were seeded in 96-well culture plates. After incubating for 24 hours, cells were treated with different concentrations of naringin (12.5, 25, 50, 100, 150, 200 and 400 µM) for 24 hours. Untreated cells were cultured in parallel as a negative control. The culture medium was removed and cells were washed with Hank’s solution (0.4 g KCl, 0.06 g KH2PO4, 0.04 g Na2HPO4, 0.35 g NaHCO3, 1 g glucose and 8 g NaCl in 1000 mL H2O). XTT test solution was diluted in culture medium without phenol red and was added to each well. After 1 hour of incubation, the absorbance was measured using a microplate reader at the wavelength of 490 nm12. The absorbance results are proportional to the percentage of viable cells. Experiments were performed in technical triplicate.
Morphological analysis
To evaluate the effects of the treatment with naringin on morphology, 5637 and T24 cells were seeded at the density of 2.0 x 105 cells/well in 12-well culture plates and incubated for 24 hours. Afterwards, cells were treated with naringin (150 and 200 µM) for 24 hours. Cells were observed and photographed using an inverted phase-contrast microscope at 200 x magnification13. Experiments were performed in technical triplicate.
Clonogenic assay
For evaluating the long-term effects of naringin, the cells were plated at a density of 1.0 × 106/25 cm3 culture flasks. After 24 hours, cells were treated with naringin (100, 150, 200, and 400 µM) and were incubated for 24 hours. Subsequently, cultures were rinsed with Hanks’ solution and the cells were removed using trypsin. Cells were plated into 12-well culture plate at 1.0 × 103 cells/well and allowed to grow for 10 days to form colonies. Afterwards, the cells were fixed with 10 % formaldehyde and stained with 0.5 % crystal violet. Absorbance was measured using a microplate reader at 560 nm wavelength11. Experiments were performed in technical triplicate.
Cell cycle analysis
To analyse the cell cycle progression after the treatment with naringin, cells were seeded at the density of 2.0 x 105 cells/well in 12-well culture plates and incubated for 24 hours. Cells were treated with naringin (100, 150, 200, and 400 µM) for 24 hours. After the treatment, cells were washed with Hank’s solution, trypsinized and centrifuged at 1,000 rpm for 10 minutes. The cell pellet formed was fixed with 70 % ethanol and maintained at -20 ºC for 12 h. Subsequently, cells were washed with Hank’s solution, centrifuged and resuspended in 200 μL of labelling solution (0.0914 g of magnesium chloride; 0.0774 g of sodium citrate; 0.04766 g of Hepes; 10 µL of Triton-X, 500 µL of propidium iodide, and 9490 µL of water). Cells were placed on ice and incubated protected from light for 30 minutes. The percentages of cells in the different cell cycle phases were measured using flow cytometry (BD FACSCalibur) and analysed using FlowJo® software14. Data from 30,000 cells were collected in each file. Experiments were performed in technical triplicate.
Wound healing assay
To assess the influence of the treatment with naringin on cell migration, the wound healing assay was performed. Cells were seeded at the density of 4.0 x 105 cells/well in 12-well culture plates. After 24 hours, the confluent cell monolayer was scraped with 200 µl sterile pipette tip to create a wound. The wound was photographed using an inverted phase-contrast microscope at 40x magnification. Afterwards, cells were treated with naringin (100, 150, 200, and 400 µM) for 24 hours. Finally, cells were again observed and photographed15. Cell migration was quantified through Image J® software by measuring the wound width in three random selected microscopic fields.
Cytokinesis-block micronucleus assay and nuclear division index (NDI)
The micronucleus assay with cytokinesis blockage was performed to assess the mutagenic potential of the treatment with naringin. Cells were plated at the density of 1.0 x 106 cell/plate in Petri dishes and incubated for 24 hours. Cells were treated with naringin (100, 150, 200, and 400 µM) for 4 hours and with doxorubicin (0.4 g/ml) for 2 hours as a positive control. After the treatments, cells were washed with Hank’s solution, cultured with 15 µL of cytochalasin B (2 mg/mL) and incubated for more 24 hours. Afterwards, cells were trypsinized, hydrated with KCl 0.075 M and fixed on microscope slides with methanol and acetic acid. The microscope slides were then stained with 5 % Giemsa. One thousand binucleated cells were analyzed in each slide to determine the frequencies of micronucleated cells. Five hundred cells were analyzed in each slide to determine the NDI. The NDI was calculated as previously described by Fenech16: NDI = M1 + 2 (M2) + 3 (M3) + 4 (M4) / N, whereas M1-M4 is the number of cells with 1-4 nuclei and N is the total number of viable cells.
Results
Quantitative structure-activity relationship (QSAR) analysis
The QSAR analysis showed that narigin presents high potential to act as anticarcinogenic, antineoplastic, and antimetastatic. The mechanisms of action with high potential are related to apoptosis (caspase 3 stimulant, caspase 8 stimulant, and apoptosis agonist), cytostatic effects and Myc inhibition. In addition, inhibition of DNA synthesis and expression inhibition of RELA and MMP9 genes were predicted as moderate potential (Table 1).
Table 1. Quantitative Structure-Activity Relationship (QSAR) analysis for potential antitumor activities of naringin using the PASS online tool
Activities | Pa - Pi value |
---|---|
Anticarcinogenic | 0.984 |
Caspase 3 stimulant | 0.892 |
Antineoplastic | 0.852 |
Cytostatic | 0.805 |
Caspase 8 stimulant | 0.727 |
Apoptosis agonist | 0.713 |
Myc inhibitor | 0.586 |
Antimetastatic | 0.507 |
DNA synthesis inhibitor | 0.479 |
RELA expression inhibitor | 0.303 |
MMP9 expression inhibitor | 0.229 |
DNA repair enzyme inhibitor | 0.114 |
P-glycoprotein inhibitor | 0.102 |
DNA intercalator | 0.039 |
Pa: potential to be active; Pi: potential to be inactive; Pa-Pi < 0,2: Low potential; Pa-Pi ≥ 0.5: Moderate potential; Pa-Pi ≥ 0.5: High potential
Cytotoxicity and cell morphology
The cytotoxicity results are show in Figure 2-a. Significant reduction in cell viability was observed in 5637 cells after treatment with 100, 150, 200, and 400 µM. In T24 cells, significant reduction in cell viability was observed at 150, 200, and 400 µM of naringin.
The evaluation of cell morphological alterations caused by the treatment with naringin shows cellular debris and digitiform projections on both cell lines tested, as can be observed in Figure 2-b.
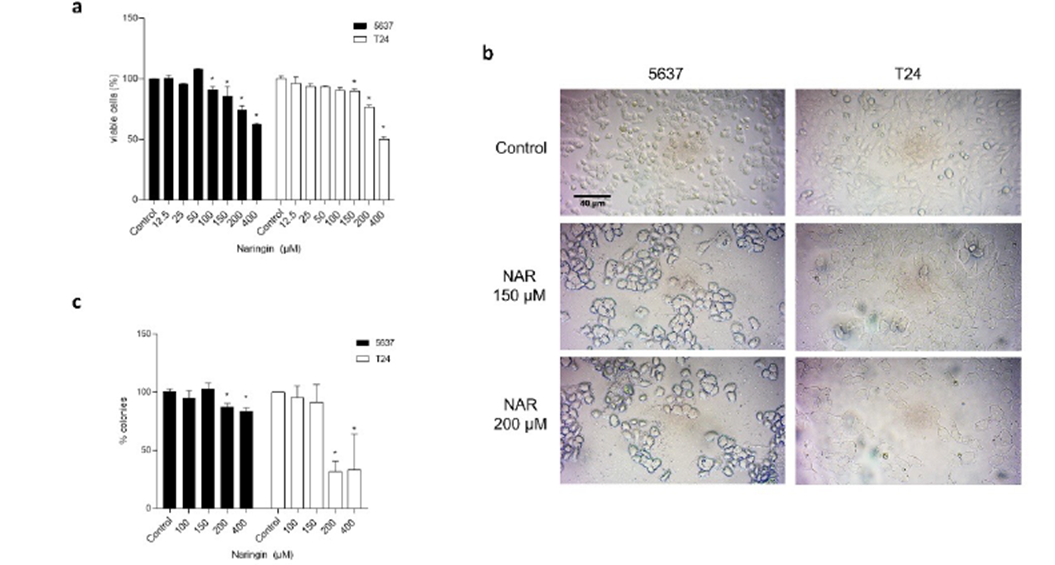
Figure 2 a) Percentages of viable 5637 and T24 cells after 24-h treatment with naringin. Control: untreated cells. *p < 0.05 compared with negative control. Each point represents the mean value obtained from three independent experiments. b) Photomicrographs of 5637 and T24 cells after 24-h treatment with naringin. Inverted phase-contrast optical microscope, 200x magnification. Control: untreated cells. NAR: naringin. c) Percentages of 5637 and T24 cell colonies after 24-h treatment with naringin. Control: untreated cells. *p < 0.05 compared with negative control. Each point represents the mean value obtained from three independent experiments.
Clonogenic survival
The results of clonogenic survival assay are presented on Figure 2-c. As can be observed, the long-term proliferation was changed after treatment with 200 µM and 400 µM for both cells.
Cell cycle progression
The results of the cell cycle analysis are shown in Figure 3. In 5637 cells, there was a significant increase in the number of cells in G0/G1 phase, followed by a significant reduction of cells in S and G2/M phases. In T24 cells, there was a significant increase in the number of cells in G2/M phase after treatment with the highest concentration of naringin.
Cell migration
The results of cell migration assay are presented in Figure 4. Naringin inhibited cell migration in both cell lines.
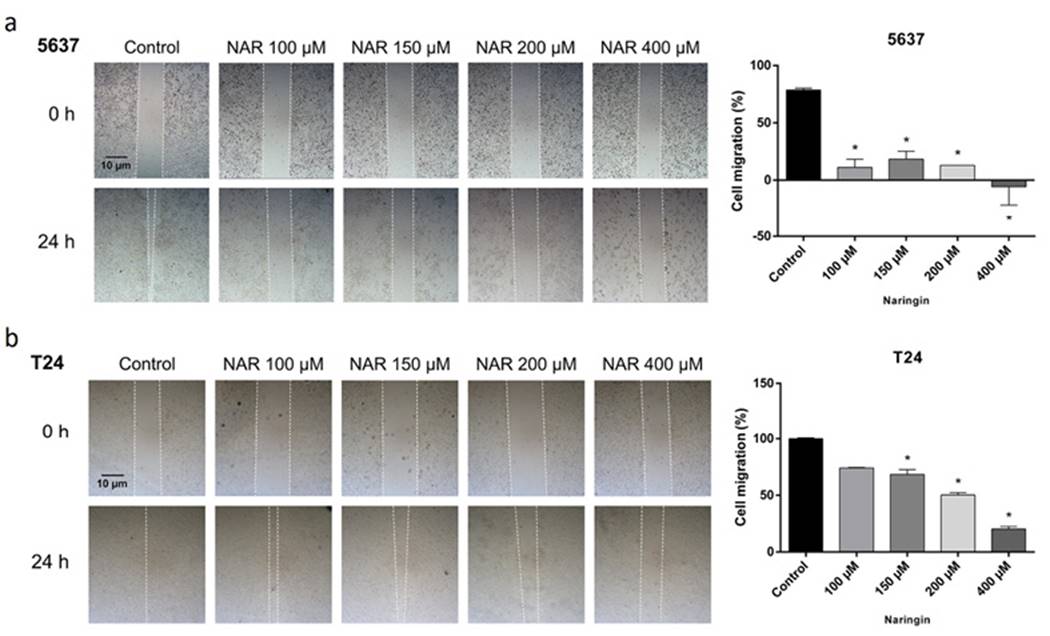
Figure 4. Photomicrographs and quantification of cellular migration of (A) 5637 and (B) T24 cells after 24-h treatment with naringin. Control: untreated cells. NAR: naringin. *p < 0.05 compared with negative control. Each point represents the mean value obtained from three independent experiments. Inverted phase-contrast optical microscope, 40x magnification.
Micronucleus assay and nuclear division index (NDI)
Narigin treatment did not cause an increase in the frequency of micronuclei (Table 2). In T24 cells, there was a significant decrease in NDI after treatment with narigin at the highest concentration (Table 3).
Table 2. Frequencies of micronucleated (‰) cells after treatment with naringin
Control | Positive Control | Naringin 100 µM | Naringin 150 µM | Naringin 200 µM | Naringin 400 µM | |
---|---|---|---|---|---|---|
5637 | 0.004 ± 0.001 | 0.014 ± 0.002* | 0.003 ± 0.002 | 0.004 ± 0.002 | 0.004 ± 0.001 | 0.005 ± 0.001 |
T24 | 0.008 ± 0.001 | 0.015 ± 0.002* | 0.006 ± 0.001 | 0.009 ± 0.001 | 0.008 ± 0.002 | 0.006 ± 0.001 |
Control: untreated control. Positive control: doxorubicin (0.4 µg/mL). p < 0.05 compared to control.
Table 3. Nuclear division index (NDI) for the 5637 and T24 cells after the treatment with naringin
Control | Naringin 100 µM | Naringin 150 µM | Naringin 200 µM | Naringin 400 µM | |
---|---|---|---|---|---|
5637 | 1.721 ± 0.014 | 1.705 ± 0.031 | 1.697 ± 0.036 | 1.707 ± 0.018 | 1.668 ± 0.010 |
T24 | 1.830 ± 0.022 | 1.854 ± 0.024 | 1.809 ± 0,037 | 1.793 ± 0.014 | 1.751 ± 0.028* |
Control: untreated control. p < 0.05 compared to control.
Discussion
We conducted this study aiming to explore the antitumor effects of naringin and its mechanism of action on bladder cells. The use of in silicostudies are interesting tools for the drug discovery process, especially to understand more about poorly studied compounds in a fast and low-cost process17.
According to in silico analysis, the cell death caused by naringin in bladder cancer cells observed in the cytotoxicity assay might occur through apoptosis. In addition to the high probability to act as an apoptosis agonist, naringin also presents a high probability to stimulate caspase-8 and caspase-3. Caspase-8 is characterized as an enzyme that initiates the extrinsic apoptotic cell death pathway induced by the activation of death receptors18. Caspase-3 acts as an effector caspase, cleaving several substrates that cause the morphological and biochemical changes seen in apoptotic cells19. Indeed, in hepatocellular carcinoma cells, naringin treatment caused an increase in caspase-3 and caspase-8 activities20. In addition, the morphological changes observed in T24 and 5637 cells are also associated with the apoptosis process21,22.
The in silico predicted moderate potential to inhibiting DNA synthesis was confirmed by clonogenic survival assay that determines the ability of a cell proliferates indefinitely, retaining its reproductive capacity after being exposed to a substance23. Clonogenic survival the most appropriate endpoint for measuring cell death in tumor cell lines24.
The naringin effect on cell cycle arrest corroborated the prediction of a high probability of naringin acting as a cytostatic agent. Several other studies also demonstrated the ability of naringin to modulate cell cycle progression25,26,27. Naringin effects on cell cycle seem to be related to modulation of the complexes of cyclins, cyclin-dependent kinases, and cyclin-dependent kinases inhibitors8, in addition to inhibiting c-MYC expression28,29.
Also confirming the in silico prediction of antimetastatic effect, naringin inhibited cell migration in bladder cancer cells. Similar effects of naringin have been observed in other types of cancer30,31. Tan et al.32 suggested that the anti-metastatic properties of naringin seem to be associated with the downregulation of matrix metalloproteinases. Indeed, the in silico results suggested naringin ability to inhibit MMP9 expression, a matrix metalloproteinase with crucial role in cancer progression33. Additionally, there is a moderate probability to naringin inhibit the RELA gene expression, which encodes the protein p65 that is upregulated in bladder cancer and is associated to cell migration34.
Regarding to micronucleus assay, treatment with naringin was not capable of inducing mutagenicity to neither of the cell lines tested. In human lymphocytes, naringin also did not induce micronucleus formation35, showing that this compound has not clastogenic or aneugenic potential.
In conclusion, our study corroborates the antiproliferative potential of naringin on bladder tumor cells and shows cytotoxicity, damage in the reproductive capacity, interference in the cell cycle progression, and alteration in cell migration by mechanisms unrelated to aneugenic or clastogenic activity. The in silico analysis showed that the mechanisms of action could be related to apoptosis (caspase 3 stimulant, caspase 8 stimulant, and apoptosis agonist) and Myc protein and RELA and MMP9 gene inhibition.