INTRODUCTION
The bone is a dynamic tissue which is under constant remodeling. Indeed, bone remodeling is a complex cellular process that involves bone resorption induced by osteoclasts and bone formation produced by osteoblasts (1). An imbalance in this equilibrium results in metabolic bone diseases such as osteoporosis or osteopetrosis. This disequilibrium may be produced by an increase in bone resorption due to a rise in the number of osteoclasts or in their activity, or by a decrease in bone formation due to a lower osteoblast activity, or by both combined effects.
The direct cellular interactions between osteoblasts and osteoclasts, mediated in part by the receptor activator of NF-κB, its ligand and osteoprotegerin (RANK/RANKL/OPG) pathway, are essential for the regulation of bone remodeling (2). The interaction between RANKL, either at the osteoblast surface or in its soluble form and its receptor RANK, on the membrane of osteoclast precursors initiates a cascade of signaling events, resulting in their differentiation to form mature osteoclasts. OPG, an osteoblast-secreted glycoprotein of the tumor necrosis factor receptor superfamily, acts as a decoy receptor and blocks the interaction between RANKL and RANK. Moreover, many other cytokines and hormones have been found to regulate either OPG or RANKL, or both, in similar or opposite directions (3).
Osteoclasts represent 1-2 % of the total bone resident cells. They are terminally differentiated giant multinucleated cells, derived from the fusion of mononuclear progenitors of the monocyte/macrophage hematopoietic lineage (1). The importance of osteoclasts in bone homeostasis is evidenced by the diseases in which osteoclast formation or function is unbalanced. However, the role of osteoclasts in health or disease and its biology have remained elusive for years. Initially, it was thought that osteoclasts were cells that undergo apoptosis after a short lifespan of around two weeks, but in the last few years, it has been shown that osteoclasts have a lifespan of around 6 months. A recent study has revealed that mature osteoclasts are capable of fissioning into smaller daughter cells, a new cell type called osteomorphs (4). These osteomorphs are freely motile cells, able to migrate and fuse with other osteomorphs or osteoclasts, creating recycled cells (4).
Osteoclasts can be studied in vitro by isolating primary bone marrow or peripheral blood monocytes cells (PBMCs), or by using the murine myeloid cell line RAW 264.7, which can be differentiated into mature osteoclasts (5-7). The use of established cell lines like RAW 264.7 instead of human primary cell cultures is extensive because it is not feasible to cultivate and expand osteoclasts for long periods of time. In addition, available cell numbers from single differentiation experiments are limited and experimental outcome may be variable depending on the cell donor. Therefore, the use of the RAW 264.7 cell line, which is quite extended, avoids these problems.
In the literature, the differentiation process of osteoclasts from RAW 264.7 cells is well established. However, the differentiation process of osteoclasts from human PBMCs is not clearly described. Thus, in scientific reports there is no defined cell density or time required to achieve the desired osteoclasts. We needed much bibliographic research in the first place, and then we performed different technical approaches to obtain osteoclasts until suitable differentiation results were achieved. All of these tasks required up to a month for the process to be completed.
Therefore, the aim of this study was to optimize osteoclast differentiation techniques, from both RAW 264.7 cells and peripheral blood monocytes. The goal was to obtain differentiated osteoclasts in order to perform experiments with substances that act on the viability and apoptosis of osteoclasts.
MATERIAL AND METHODS
MATERIALS
Alpha-minimum essential medium (aMEM), Dulbecco’s modified Eagle medium (DMEM), fetal bovine serum (FBS), Phosphate Buffered Saline (PBS), l-glutamine and trypsin were purchased from Invitrogen (Thermo Fisher Scientific) (Waltham, MA, USA); accutase and toluidine blue were purchased from Sigma Chemical Co. (St. Louis, MO, USA); penicillin-streptomycin from LabClinics (Barcelona, Spain); recombinant mouse RANKL, recombinant human RANKL and recombinant human M-CSF from R&D systems (Bio-Techne, UK); RosetteSep™ Human Monocyte Enrichment Cocktail and Lymphoprep™ were purchased from Stemcell Technologies (Köln, Germany).
OSTEOCLASTS FROM RAW 264.7 CELL CULTURE
RAW 264.7 cells, a transformed murine monocytic macrophage cell line from the European Collection of Authenticated Cell Cultures (ECACC, England), were cultured at 37 °C in 5 % CO2 atmosphere in DMEM, supplemented with 10 % heat-inactivated FBS and 100 U/mL penicillin-streptomycin. Cells were plated directly on 96-well plates at a density of 1.5 x 104, 2.5 x 104 and 3.5 x 104 cells/cm2. The medium used for osteoclastic differentiation was aMEM supplemented with 10% heat-inactivated FBS, 100 U/mL penicillin-streptomycin and 25, 30 and 50 ng/mL of recombinant mouse RANKL. The medium was removed and replaced with fresh medium every 48 h-72 h. After 6 days, RAW 264.7 cells were differentiated into osteoclasts.
OSTEOCLASTS FROM PERIPHERAL BLOOD MONOCYTE CULTURE
RosetteSep™ Human Monocyte Enrichment Cocktail™ was used to purify human monocytes taken from total PBMCs of buffy coats, supplied by the local reference blood bank (Banc de Sang i Teixits, Barcelona). Cells were separated using Lymphoprep™, following the manufacturer’s protocol.
Cells were seeded in two different ways. For the first attempt, they were seeded in a 75 cm2 flask at a density of 150,000 cells/cm2, and then cultured at 37 °C in 5 % CO2 atmosphere in aMEM, supplemented with 10 % heat-inactivated FBS, 100 U/mL penicillin-streptomycin and 25 ng/mL of recombinant human macrophage colony-stimulating factor (M-CSF) for 2 weeks. The medium was replaced every 48 to 72 hours. Then, cells were treated with accutase and plated on 96-well plates at a density of 80,000 cells/cm2 for one additional week, adding 30 ng/mL of recombinant human RANKL to the medium. Human monocytes were differentiated into osteoclasts. On the other hand, cells were seeded directly on 96-well plates at a density of 150,000, 250,000 and 400,000 cells/cm2 and were treated from the beginning with both 25 ng/mL of recombinant human M-CSF and 30 ng/mL of recombinant human RANKL in aMEM supplemented with 10 % heat-inactivated FBS, 100 U/mL penicillin-streptomycin for three-four weeks.
CHARACTERIZATION OF OSTEOCLASTS AND RESORPTION PIT FORMATION ASSAY
To identify the generation of multinucleated osteoclasts, cells were stained for the enzyme tartrate-resistant acid phosphatase (TRAP) using the TRAP-staining kit (Sigma-Aldrich, St. Louis, MO, USA), according to the manufacturer’s instructions. TRAP-positive multinucleated (3 or more nuclei) cells were visualized by light microscopy. Each osteoclast characterization assay was performed at least 3 times.
Resorption pits were assessed by seeding RAW 264.7 cells to differentiate into osteoclasts at a density of 25,000 cells/cm2 on 24-well Corning® Osteo Assay Surface Plates (Corning Cat. No. 3987, Cultek, Life Sciences, NY, USA). Cells were then removed and well surfaces were stained with 1 % toluidine blue for 1 min. Individual resorption pits or multiple pit clusters were visualized on the surfaces by light microscopy.
Images were obtained using a DM IL LED-inverted microscope with a MC190 HD camera and the Application Suit v3.4.0 acquisition software (Leica microsistemas SLU, Spain). The area of pits was analyzed using Image J software (NIH, Bethesda, MD, USA).
RESULTS
DIFFERENTIATION AND IDENTIFICATION OF OSTEOCLASTS
The optimal cell density for RAW 264.7 differentiation cells was 25,000 cells/cm2. A concentration of 30 ng/mL of RANKL was enough to achieve an optimal differentiation. Under this condition, multinucleated osteoclasts were observed by TRAP staining after 5-7 days (Fig. 1). Osteoclastic ruffled border and connections between multinucleated osteoclasts were observed as well (Fig. 1). However, we obtained no differentiated osteoclasts when monocytes were plated directly on 96-well plates, being the method by which we differentiated RAW into osteoclasts. By contrast, in the case of monocytes from PBMCs, the cells remained monocyte-like after 3-4 weeks on 96-well plates and differences in the doses of RANKL and M-CSF did not change this result. We did not differentiate the cells by putting glass coverslips on the wells, as recommended by some research groups, since from our perspective, this technical approach did not bring advantages and made the methodology more complex. For this reason, we opted to maintain the cells in 75 cm² flasks for the time being by administering only M-CSF to keep the cells in a resting and stable state. After 3 weeks, we finally transferred the cells to 96-well plates and started administering RANKL as well. In this manner, we were able to observe differentiated osteoclasts within another week. In the differentiation process into osteoclasts from PBMCs, multinucleated osteoclasts were observed by TRAP staining after 3-4 weeks, as shown in figure 2.
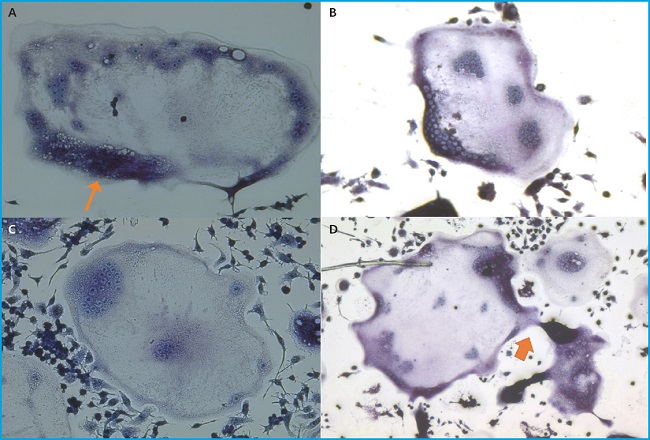
Figure 1. Differentiated osteoclasts from RAW 264.7 cells are shown after TRAP staining. Big multinucleated osteoclasts are shown. Ruffled border (1A, thin arrow) and connections between multinucleated osteoclasts (1D, thick arrow) are observed. Experiments were performed more than 10 times.
RESORPTION PITS
Individual pits or multiple pit clusters were observed using a microscope at 10x magnifications in the Corning® Osteo Assay surface plates (Fig. 3). When activators of osteoclast activity such as bilirubin were added, more resorbed areas were observed compared to the non-treated plates (data not shown). Indeed, we found that bilirubin was an activator of osteoclast survival in our viability studies, resulting in an increase in osteoclast number and activity, identified by observing the resorption pits on the Corning® Osteo Assay surface plates.
DISCUSSION
Osteoclasts are fascinating cells for their abilities and functions. Their tight balance with osteoblasts is regulated by multiple factors that make a very accurate process. With the recent discovery of osteomorphs by McDonald et al. (4), this process has turned out to be even more precise. Osteomorphs will remain waiting until the moment in which mature osteoclasts are required to resorb bone.
In our experience, the differentiation process of the cells is not free of technical difficulties. Several factors could act on this process, and osteoclasts may not become active, or simply not correctly differentiated. The differentiation of osteoclasts from RAW 264.7 cells was easier than with primary human cells, because it was a simpler process with more cell availability, and in addition, the time to achieve differentiated cells was shorter than with primary cells. We found several bibliographic references as a guide to begin with and we found our optimal concentrations of both RANKL and RAW 264.7 cells in a short time. However, the differentiation of osteoclasts from monocytes of human PBMCs was complex. First, the heterogeneity that we found in the literature was high, and it was difficult to decide how to start our experiments. Secondly, monocytes take a long time to become osteoclasts. Moreover, the diffentiation process was more expensive than using RAW cells because in this case, two differentiating activators were needed: the human M-CSF and RANKL. Despite all these setbacks, after several months, we were able to obtain active differentiated osteoclasts and we performed the experiments shown in our recent publication (8). We don’t know the reason why finally we needed to maintain for 2 weeks the monocytes from PBMCs in flasks with M-CSF. Our hypothesis is that cells maybe need a rest time to stabilize in the flask, where there is more place for them. After that period, they are able to differentiate into osteoclasts with the addition of RANKL.
The possibility of observing the activity of osteoclasts on special bone surfaces was described in a few reports (9). The observation of resorbed areas in the Corning® Osteo Assay Surface Plates allows us to check the ability of the mature osteoclasts to resorb mineralized tissue. Although we have not shown these results, we performed a study in which we administrated substances that enhance or inhibit osteoclast activity, and the resorbed areas varied to a large extent. We intended to repeat these experiments several more times, but these plates were no longer manufactured.
In summary, we have established optimized conditions for osteoclast differentiation from both cell types: the RAW 264.7 murine cell line and from human monocytes. This experience will enable us and other researchers to carry out future studies with osteoclasts.