Mi SciELO
Servicios Personalizados
Revista
Articulo
Indicadores
-
Citado por SciELO
-
Accesos
Links relacionados
-
Citado por Google
-
Similares en SciELO
-
Similares en Google
Compartir
Revista de Osteoporosis y Metabolismo Mineral
versión On-line ISSN 2173-2345versión impresa ISSN 1889-836X
Rev Osteoporos Metab Miner vol.15 no.1 Madrid ene./mar. 2023 Epub 29-Mayo-2023
https://dx.doi.org/10.20960/revosteoporosmetabminer.00007
ORIGINALS
The secretome of mechanically stimulated osteocytes modulates mesenchymal cell function
3Institute of Applied Medicine of the Universidad San Pablo-CEU. Madrid, Spain
4Department of Basic Medical Sciences. School of Medicine. Universidad San Pablo-CEU. CEU Universities. Madrid, Spain
The skeleton is a metabolically active organ that is continuously remodeled throughout our lives. This remodeling entails a balance between the formation of bone conducted by the osteoblasts and resorption by osteoclasts. Osteocytes regulate these two processes and their mechanical stimulation is essential to maintain the good functioning of bones and prevent diseases such as osteoporosis. Osteocyte stimulation causes an alteration in the production and secretion of signaling molecules that regulate osteoblast and osteoclast activity. Mesenchymal stem cells have been proposed as a possible cellular therapy for the regeneration of different tissues including bone tissue. We hypothesize in the present study that the secretome of mechanically stimulated osteocytic mouse cells affect the proliferative, adhesive capacity and gene expression of undifferentiated mesenchymal cells and preosteoblastic mesenchymal cells. To that end, the above-mentioned biological processes were analyzed in continuous preosteoblastic cellular lines and mouse mesenchymal cells in the presence of the medium conditioned by MLO-Y4 osteocytic cells after undergoing a mechanical stimulus by fluid flow. It was observed that proliferation increased in both cellular lines in the presence of secretome of mechanically stimulated osteocytes versus control while non-mechanically stimulated osteocytes caused its reduction. It was also possible to observe an increased adhesive capacity of C3H/10T1/2 cells after stimulation with the secretome of mechanically stimulated osteocytes. Regarding gene expression, only the adipogenic factor PPARᵞ underwent alterations in MC3T3-E1 cells by the secretome of osteocytes. These studies indicate that osteocytes can modify the biological behavior of mesenchymal cells by the secretion of soluble factors.
Keywords: Mechanical stimulus; Osteocytes; Mesenchymal cells; Mechanotransduction
INTRODUCTION
The skeleton is a metabolically active organ that is continuously remodeled throughout our lives. The bone remodeling process, in which bone matrix resorption and formation are coupled, serves the purpose of adjusting bone architecture to the mechanical needs of the bone. In addition, it helps repair damage to the bone matrix and prevent the accumulation of old bone that may have lost its mechanical properties (1).
There are many factors that modulate the formation and maintenance of our skeleton. Among them, mechanical load represents the main extrinsic factor with direct effects on bone tissue (2). Load on our bones translates into mechanical tensions that are received by bone cells and transformed into biological signals, which is known as mechanotransduction (2).
Osteoblasts and osteoclasts are the cells involved in bone formation and resorption, respectively (3). Osteocytes, the most abundant bone tissue cells of all, are the main receptors of mechanical stimulation and capable of communicating with osteoblasts and osteoclasts by modulating their activity (4).
Bone cells are in close contact with the cells present in the bone marrow. There we find a very heterogeneous cell population where hematopoietic cells coexist giving rise to different blood populations with the bone marrow microenvironment for non-hematopoietic cells such as perivascular and mesenchymal cells (MSC) among others (5).
The MSC present in the marrow are the source of osteoprogenitor cells that make it possible for fractures to be repaired and new bone to be formed (5).
The differentiation of mesenchymal stem cells is a process that consists of 2 stages; commitment to a cellular lineage (from the MSC to a progenitor of a specific lineage) and maturation (from progenitors to a specific cellular type). A large number of signaling pathways are involved in the regulation of MSC lineage commitment, among which we can find the TGF-beta family, BMP (bone morphogenic proteins), Wnt, hedgehogs (Hh), Notch, and fibroblast growth factors (FGF) (6,7).
In this article we study the communication between osteocytes and mesenchymal cells, and how mechanical stimulation modifies the proliferation, adhesion and gene expression of mesenchymal cells through osteocytes.
We hypothesized that the secretome of mechanically stimulated osteocytes induces biological processes related with the strengthening of bone regeneration capabilities of mesenchymal cells, and for that purpose we have studied the effect of the medium conditioned by mechanically stimulated MLO-Y4 osteocytes (by fluid flow) or not (static control) on different biological processes of C3H/10T1/2 mesenchymal and MC3T3-E1 preosteoblastic cells.
MATERIAL AND METHODS
CELL CULTURES
The following cellular lines were used for the assays of the present work: mouse mesenchymal cells uncommitted to osteoblastic lineage (C3H/10T1/2 subclone 8, ATTC, CCL-226). These cells were cultured with BME medium (“basal eagle medium,” Thermofisher, 21010046) + 10 % fetal bovine serum (FBS) (Thermofisher, 10500064) + 1 % L-glutamine (Thermofisher, 25030024) + 1 % penicillin/streptomycin (Thermo- fisher, 15140122) at 37 °C with 5 % of CO2. Mouse preosteoblastic mesenchymal cells (MC3T3-E1 subclone 4, ATTC, CRL-2593). These cells were cultured with MEM alpha medium (“minimum essential medium,” Thermofisher, A1049001) + 10 % FBS + 1 % L-glutamine and 1 % streptomycin/penicillin at 37 °C with 5 % of CO2. Murine MLO-Y4 osteocytic cells (Kerafast, EKC002). These cells were cultured with MEM alpha medium without ascorbic acid (Thermofisher, 22571038) + 2.5 % FBS + 2.5 % of calf serum (“calf serum” [CS], Thermofisher, 16010159) and 1 % streptomycin/ penicillin at 37 °C with 5 % of CO2.
MECHANICAL STIMULATION BY FLUID FLOW
A collection of 250 000 MLO-Y4 cells were seeded on Teflon-coated glass slides in a 15 cm2 area previously covered with a collagen matrix (Sigma Aldrich, 000010C8919). After 48 hours, the cells unerwent a mechanical stimulus (FF) using the Flexcell Streamer fluid shear stress device that produces a stress of 10 dynes/cm2 for 10 minutes (Flexcell International Corporation, Hillsborough, North Carolina, USA). As static control or SC, MLO-Y4 cells were seeded on a Teflon-coated glass slide without undergoing any mechanical stimulus. Afterwards, the cells were incubated for 18 hours, with MEM alpha medium without phenol red (Gibco) supplemented with 0.5 % of CS, 0.5 % of FBS, and 1 % of penicillin-streptomycin to obtain conditioned media (CM) of the different experimental groups: mechanostimulated cells (FF) and control cells.
PROLIFERATION ASSAY
To conduct proliferation assays, both MC3T3-E1 and C3H/10T1/2 cell lines were seeded at a density of 20 000 cells/well in 24-well culture microplates (VWR,734-2325). The following day the medium was changed for a medium with 250 µl of conditioned medium (static control or fluid flow) and 750 µl of its corresponding culture medium. MC3T3-E1 and C3H/10T1/2 cells were used as control incubated with 250 µL of MEM alpha without phenol red (Gibco) supplemented with 0.5 % of CS, 0.5 % of FBS, 1 % of penicillin-streptomycin, and 750 µl of its corresponding culture medium. After 24 h of incubation the cells were detached with Trypsin-EDTA (Thermofisher, 25300062) and cell count started with Trypan blue (Thermofisher, 15250061) in Neubauer chamber. The same process was repeated at 48, 72 and 96 hours and assessment of the proliferation was obtained.
CELL ADHESION
Adhesion of MC3T3-E1 preosteoblastic cells and C3H/10T1/2 mesenchymal cells was evaluated by cell quantification of marked with vital fluorescent dye cytopainter (Abcam, ab176735). To that end, MC3T3-E1 or C3H/10T1/2 cells were seeded in P6 plates for 24 hours. The following day the medium was changed for a medium with 250 µl of the conditioned medium (static control or fluid flow) and 750 µl of its corresponding culture medium. MC3T3-E1 and C3H/10T1/2 cells were used as control of the experiment and incubated with 250 µL of MEM alpha without phenol red (Gibco) supplemented with 0.5 % of CS, 0.5 % of FBS, 1 % of penicillin-streptomycin, and 750 µl of its corresponding culture medium. After 24 hours, they were incubated with cytopainter for 30 minutes and, after incubation, the cells were lifted, counted, and a total of 20 000 cells/cm2 were seeded on a new P6 well for 30 minutes. Finally, the supernatant was collected and the cells that were not adhered were counted in a Neubauer chamber using the exclusion method with Trypan blue. On the other hand, images of the cells adhered to the well surface were obtained through a PAULA microscope (“Personal automated lab assistant, Leica Microsystems”). The number of cells marked with fluorescence was counted in 3 different, random fields.
REAL-TIME POLYMERASE CHAIN REACTION (RT-PCR)
A collection of 300 000 cells were seeded in each well in 6-well culture plates in the case of MC3T3-E1 preosteoblastic cells, and 450 000 in the case of C3H/10T1/2 mesenchymal cells. After 24 hours of incubation, the cells were washed with PBS and then PBS was replaced by 250 µl of MEM alpha in the controls and 250 µl in the corresponding conditioned medium in the other wells. In addition, each well was added 750 µl of its corresponding medium. After 24 hours of incubation the culture medium was removed, each medium was collected from the wells in 1.5 mL tubes using trizol and stored at -80 °C.
The following day the samples were defrosted, and kept in ice to maintain a low temperature and then the RNA extraction protocol was performed. Reverse transcription to obtain cDNA was performed with the cDNA reverse transcription kit (“High-capacity RNA-to-cDNA Kit”, Thermofisher, 4387406) following the instructions. After the cDNA was obtained, PCR was performed in a P384 plate using the “Sybergreen” (TB Green, Condalab RR420A) to study the following genes: RUNX2, alkaline phosphatase (ALP), PPARγ, and osteocalcin. In addition, beta actin (cytoskeletal actin) was used as constitutive gene expression. To that end, an ABI PRISM 7500 thermocycler was used (Applied Biosystems).
STATISTICAL ANALYSIS
Data are expressed as means ± standard error. The distribution of the data was analyzed and since they did not adjust to a normal distribution, the differences among the experimental groups were evaluated by non-parametric variance analysis (Kruskal-Wallis). Determination of the possible differences among the experimental groups was conducted using the Dunn or Mann-Whitney tests. To conduct the statistical analysis the software GraphPad Prism was used. p values < 0.05 were considered statistically significant.
RESULTS
EFFECTS OF THE CONDITIONED MEDIUM OF MECHANICALLY STIMULATED —OR NOT— OSTEOCYTES ON THE PROLIFERATION OF MESENCHYMAL CELLS AND PREOSTEOBLASTS
Proliferation study of mouse MC3T3-E1 preosteoblastic cells and mesenchymal cells (C3H/10T1/2) was conducted with 25 % of conditioned medium of MLO-Y4 mouse osteocytic cells mechanically stimulated (FF) or not (SC) (Fig. 1).
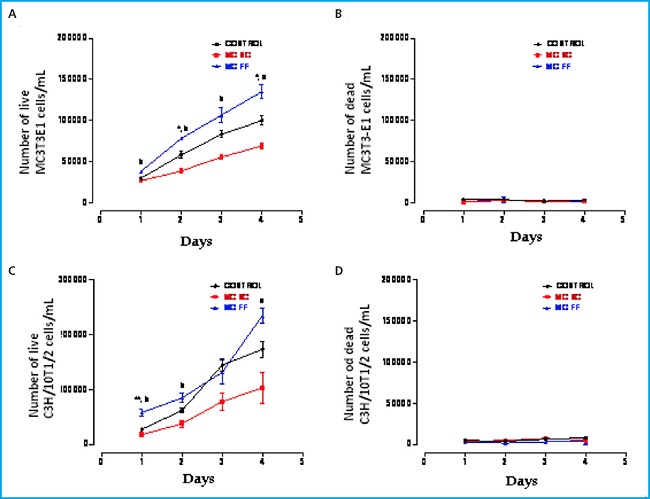
Figure 1. Proliferation and cellular death of MC3T3-E1 preosteoblastic cells (A and B, respectively) and C3H/10T1/2 embryonic mesenchymal cells (C and D, respectively) in the presence or absence of conditioned MLO-Y4 osteocyte media after mechanical stimulation (fluid flow: MC FF) or not (static control: MC SC). The controls represent cells without stimulation of osteocyte conditioned media. The values are the mean ± standard error of the 3 experiments conducted in triplicate. *p < 0.05 vs. control; **p < 0.01 vs. control; ap < 0.05 vs. MC SC; bp < 0.01 vs. MC SC.
We observed that in both cell lines the osteocyte secretomes show significant effects in proliferation. While the mechanically stimulated (FF) osteocyte secretome promotes greater proliferation, non-stimulated (SC) osteocytes secretome induces less proliferation versus control (Fig. 1 A and C). No differences were observed in cell viability in any of the three experimental conditions in the 4 days analyzed (Fig. 1 B and D).
EFFECTS OF THE CONDITIONED MEDIA OF MECHANICALLY STIMULATED —OR NOT— OSTEOCYTES ON ADHESION OF MESENCHYMAL CELLS AND PREOSTEOBLASTS
Afterwards, a mouse MC3T3-E1 preosteoblastic cell and mesenchymal cell (C3H/10T1/2) adhesion study was conducted in the presence of secretome of osteocytic MLO-Y4 mouse cells after stimulation (FF) or lack (SC) of mechanical stimulus by fluid passage.
No significant differences were seen in the adhesion of MC3T3-E1 cells in the different experimental conditions (Fig. 2). Regarding the number of unattached cells, we observed that stimulation with conditioned media (FF and SC) hardly decreased adhesion compared to control conditions (Fig. 2).
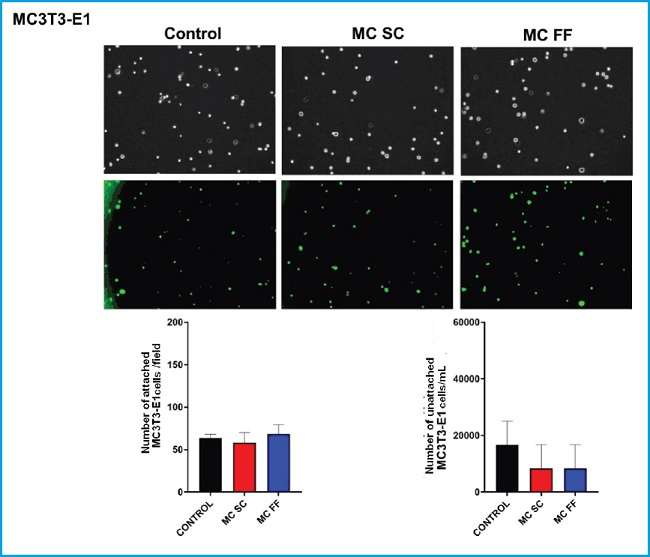
Figure 2. Adhesion of MC3T3-E1 preosteoblastic cells in the presence or absence of conditioned MLO-Y4 osteocyte media after mechanical stimulation (fluid flow: MC FF) or not (static control: MC SC). The controls represent cells without stimulation of osteocyte conditioned media. The values are the mean ± standard error of 3 experiments conducted in triplicate. Representative images are shown after being obtained through bright field microscopy (upper panel) or epifluorescence microscopy (lower panel). Results are expressed as the number of attached cells/field (lower left) and unattached cells/mL (lower right). The values are the mean ± standard error of 3 experiments conducted in triplicate.
However, we did observe a clear difference among different experimental conditions in C3H/10T1/2 cell adhesion. The medium conditioned by mechanically stimulated osteocytes increased adhesion of this cell line (Fig. 3).
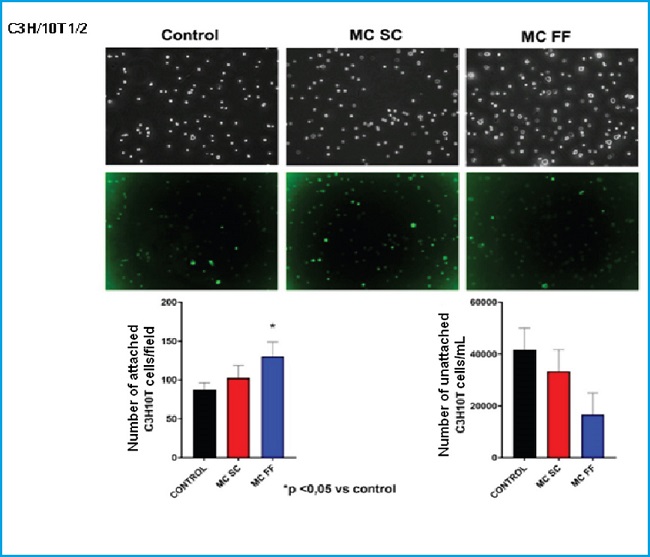
Figure 3. Adhesion of C3H/10T1/2 embryonic mesenchymal cells in the presence or absence of conditioned MLO-Y4 osteocyte media after mechanical stimulation (fluid flow: MC FF) or not (static control: MC SC). The controls represent cells without stimulation of osteocyte conditioned media. The values are the mean ± standard error of 3 experiments conducted in triplicate. Representative images are shown after being obtained through bright field microscopy (upper panel) or epifluorescence microscopy (lower panel). The results are expressed as the number of attached cells/field (lower left) and unattached cells /mL (lower right). The values are the mean ± standard error of 3 experiments conducted in triplicate. *p < 0.05 vs. control.
ANALYSIS OF GENE EXPRESSION IN MESENCHYMAL AND PREOSTEOBLASTIC CELLS TREATED WITH CONDITIONED MEDIA OF OSTEOCYTES WHETHER STIMULATED MECHANICALLY OR NOT
We conducted a study of differentiation markers and bone formation (Runx2, osteocalcin and alkaline phosphatase) and adipogenic differentiation (PPARᵞ) in mouse MC3T3-E1 preosteoblastic cells and mesenchymal cells (C3H/10T1/2) stimulated with 25 % of conditioned media of mouse MLO-Y4 osteocytic cells in the presence (FF) and absence (SC) of a mechanical stimulus by fluid flow. Figures 4 and 5 show no significant changes on the expression of Runx2, osteocalcin, alkaline phosphatase or any of the two lines studied.
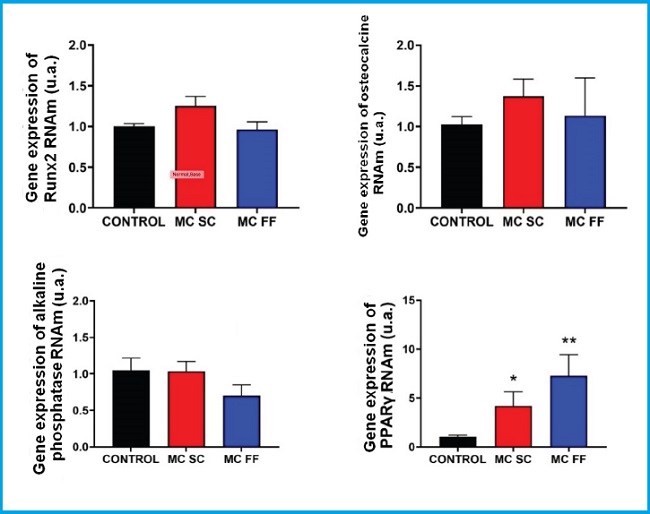
Figure 4. Gene expression analysis of bone differentiation and formation markers (Runx2, osteocalcin, and alkaline phosphatase) and adipogenic differentiation (PPARᵞ) in MC3T3-E1 preosteoblastic cells in the presence or absence of conditioned MLO-Y4 osteocyte media after mechanical stimulation (fluid flow: MC FF) or not (static control: MC SC). The controls represent cells without stimulation of osteocyte conditioned media. The values are the mean ± standard error of 3 experiments conducted in triplicate. *p < 0.05 vs. control; **p < 0.01 vs. control.
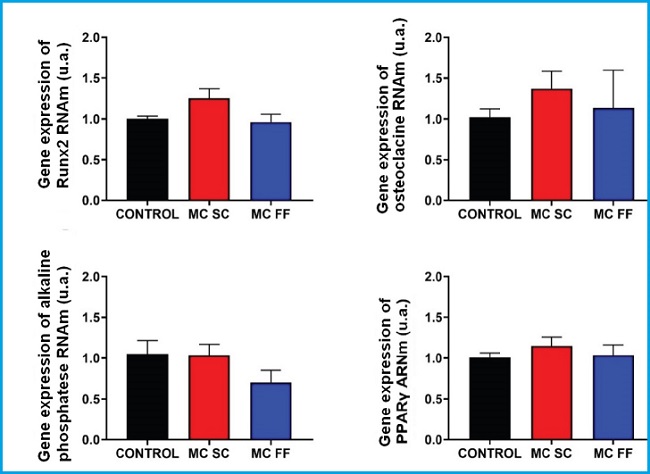
Figure 5. Gene expression analysis of bone differentiation and formation markers (Runx2, osteocalcin, and alkaline phosphatase) and adipogenic differentiation (PPARᵞ) in C3H/10T1/2 preosteoblastic cells in the presence or absence of conditioned MLO-Y4 osteocyte media after mechanical stimulation (fluid flow: MC FF) or not (static control: MC SC). The controls represent cells without stimulus of osteocyte conditioned media. The values are the mean ± standard error of 3 experiments conducted in triplicate.
However, the PPARγ expression increased after stimulation with conditioned media of osteocytes SC and FF in MC3T3-E1 preosteoblastic cells (Fig. 4) while in C3H/10T1/2 cells, the PPARγ expression levels remained practically unchanged in all the experimental conditions (Fig. 5).
DISCUSSION
Aging and certain bone diseases such as osteoporosis change bone remodeling and associated loss of bone mass. This is due to the accumulation of apoptotic osteocytes and the recruitment of osteoclast precursors that promote the bone resorption process. This situation can be prevented through mechanical stimulation since it is applied naturally on osteocytes when we’re doing exercise (8).
Former studies have already demonstrated that the conditioned medium of mechanically stimulated osteocytes can recruit osteoprogenitors (mesenchymal cells and osteoblasts), and promote commitment of osteogenic lineage of these cells to replace exhausted osteoblasts, improve bone formation, and strengthen tissues (9). In addition, mechanical stimulation plays an essential role when it comes to modulating certain cell processes. In our results, we found that exposure of MC3T3-E1 preosteoblastic cells and C3H/10T1/2 mesenchymal cells to conditioned media containing the secretome of mechanically stimulated MLO-Y4 osteocytic cells affects their proliferation and adhesion, as well as the gene expression of the markers studied. Some discrepancies in the results obtained between the two cell lines used in this study can be due to the different nature of each cell type. The MC3T3-E1 preosteoblastic cell line is at a greater degree of differentiation and commitment towards an osteoblastic phenotype compared to C3H/10T1/2 cells. The latter, because they are of embryonic origin, are more undifferentiated and possibly because of this and because of their character as embryonic stem cell, can show greater proliferation compared to more differentiated lines, such as MC3T3-E1 (10).
Regarding the differences of control cells with respect to those exposed to conditioned media, the results could indicate that mechanically stimulated in vivo osteocytes maintain bone mass levels when inducing, by secretion of molecules, proliferation of mesenchymal cells that can subsequently be differentiated from osteoblasts that will be making up the bone matrix. However, osteocytes that have not been mechanically stimulated show a modified secretome that probably implies a reduced secretion of proliferating factors that act on mesenchymal cells or increase of secretion of proliferation-inhibiting factors. In any of the two situations, the result would entail less proliferation of mesenchymal cells and possibly less osteoblast differentiation to create the bone matrix.
Because they are less differentiated cells C3H/10T1/2 mesenchymal cells could be considered to show less expression of proteins that are often present in more specialized cells. Therefore, it would be possible that, since they are not committed to a specific cellular phenotype, they can be modified more easily by the secretome of mechanically stimulated osteocytes compared to more differentiated cells like MC3T3-E1 that are already committed to the expression of proteins of osteoblastic lineage. However, the more significant effects of gene expression observed in this study after stimulation with osteocyte secretome are associated with an increased PPARγ expression in MC3T3-E1 cells. This change could be associated with more adipogenesis associated with aging, bone mass loss, and regeneration delay (11), the process opposite to osteoblastogenesis (12), and with the other results obtained in this study. However, some studies indicate that, in some stages of differentiation to osteoblasts PPARγ expression can play an important role (13,14). That is why it will be necessary to conduct more experiments to prove more directly the adipogenic and osteogenic potential of osteocyte secretome on the different types of mesenchymal cells used in this article.
Regarding cell adhesion, for more adhesion to occur an increase in the expression of matrix binding proteins like integrins is often necessary (15). It is possible that, due to overexposure of these matrix binding proteins, mesenchymal cells adhere more easily after being stimulated with mechanically stimulated osteocytes secretome. The adhesive capacity of mesenchymal cells is a necessary requirement for regeneration therapy to occur (16) because, since they are substrate-binding dependent cells, they first need to bind to the damaged areas to be able to start proliferation or differentiation processes.
Therefore, based on our own results, we can assume that certain factors of osteocyte secretome favor mesenchymal cell adhesion, and maybe regeneration processes could indirectly be promoted from these cells.
Acknowledgements:
we wish to thank the support given from the Spanish Society for Bone Research and Mineral Metabolism (SEIOMM) and the Spanish Foundation for Bone and Mineral Metabolism Research (FEIOMM) for making this manuscript possible.
REFERENCES
1. Hadjidakis DJ, Androulakis II. Bone remodeling. Ann N Y Acad Sci 2006;1092:385-96. DOI: 10.1196/annals.1365.035 [ Links ]
2. Stewart S, Darwood A, Masouros S, Higgins C, Ramasamy A. Mechanotransduction in osteogenesis. Bone Joint Res 2020;9(1):1-14. DOI: 10.1302/2046-3758.91.BJR-2019-0043.R2 [ Links ]
3. Siddiqui JA, Partridge NC. Physiological Bone Remodeling: Systemic Regulation and Growth Factor Involvement. Physiology (Bethesda) 2016;31(3):233-45. DOI: 10.1152/physiol.00061.2014 [ Links ]
4. Schaffler MB, Cheung WY, Majeska R, Kennedy O. Osteocytes: master orchestrators of bone. Calcif Tissue Int 2014;94(1):5-24. DOI: 10.1007/s00223-013-9790-y [ Links ]
5. Pierce JL, Begun DL, Westendorf JJ, McGee-Lawrence ME. Defining osteoblast and adipocyte lineages in the bone marrow. Bone 2019;118:2-7. DOI: 10.1016/j.bone.2018.05.019 [ Links ]
6. Lin GL, Hankenson KD. Integration of BMP, Wnt, and notch signaling pathways in osteoblast differentiation. J Cell Biochem 2011;112(12):3491-501. DOI: 10.1002/jcb.23287 [ Links ]
7. Wu M, Chen G, Li YP. TGF-β and BMP signaling in osteoblast, skeletal development, and bone formation, homeostasis and disease. Bone Res 2016;4:16009. DOI: 10.1038/boneres.2016.9 [ Links ]
8. Cadenas Martín M, Tirado I, Martín E, Ardura J, Bravo B, Gortazar AR. Efectos de la estimulación mecánica en la comunicación entre células óseas. Rev Osteopor Metab Miner 2019;11(1):12-8. DOI: 10.4321/S1889-836X2019000100003 [ Links ]
9. Brady RT, O'Brien FJ, Hoey DA. Mechanically stimulated bone cells secrete paracrine factors that regulate osteoprogenitor recruitment, proliferation, and differentiation. Biochem Biophys Res Commun 2015;459(1):118-23. DOI: 10.1016/j.bbrc.2015.02.080 [ Links ]
10. Ding DC, Shyu WC, Lin SZ. Mesenchymal Stem Cells. Cell Transplant 2011;20(1),5-14. DOI: 10.3727/096368910X [ Links ]
11. Kawai M, Sousa K, MacDougald O, Rosen C. Themanyfacets of PPARγ: novel insights for the skeleton. Am J Physiol Endocrinol Metab 2010;299(1):E3-E9. DOI: 10.1152/ajpendo.00157.2010 [ Links ]
12. Lee S, Choi E, Cha MJ, Hwang KC. Cell Adhesion and Long-Term Survival of Transplanted Mesenchymal Stem Cells: A Prerequisite for Cell Therapy. Oxid Med Cell Longev 2015;2015:632902. DOI: 10.1155/2015/632902 [ Links ]
13. Jackson SM, Demer LL. Peroxisome proliferator-activated receptor activators modulate the osteoblastic maturation of MC3T3-E1 preosteoblasts. FEBS Lett 2000;471(1):119-24. DOI: 10.1016/s0014-5793(00)01372-7 [ Links ]
14. Yasuda E, Tokuda H, Ishisaki A, Hirade K, Kanno Y, Hanai Y, et al. PPAR-gamma ligands up-regulate basic fibroblast growth factor-induced VEGF release through amplifying SAPK/JNK activation in osteoblasts. Biochem Biophys Res Commun 2005;328(1):137-43. DOI: 10.1016/j.bbrc.2004.12.163 [ Links ]
15. Moreno-Layseca P, Icha J, Hamidi H, Ivaska J. Integrin trafficking in cells and tissues. Nat Cell Biol 2019;21(2):122-32. DOI: 10.1038/s41556-018-0223-z [ Links ]
16. Chamberlain G, Fox J, Ashton B, Middleton J. Concise Review: Mesenchymal Stem Cells: Their Phenotype, Differentiation Capacity, Immunological Features, and Potential for Homing. Stem Cells 2007;25(11):2739-49. DOI: 10.1634/stemcells.2007-0197 [ Links ]
Received: July 22, 2022; Accepted: February 27, 2023