INTRODUCTION
Recently, the awareness of functional food’s importance is highly increasing as this food has several potential health-promoting activities. In this sense, consumers highly demand those ingredients that positively affect intestinal flora (1). Among these functional ingredients are human lactoferrin (hLf) and human milk oligosaccharides (HMOs) such as galactooligosaccharides (GOS). hLf is a 703-amino acid glycoprotein isolated from the whey fraction of human milk which exerts many biological effects such as antimicrobial, modulation of the immune system, and iron metabolism (2). Many previously published data revealed the prebiotic nature of these components of human milk, where they can improve the growth of some beneficial bacteria, i.e., Bifidobacteria and Lactobacilli (3,4). Thus, the gut microbiota of breast-fed infants is characterized by its high content of these healthy microorganism as compared to those fed infant formulas (5). Therefore, and in line with the current trend in infant formula manufacturing, several infant formulas exist in the market containing Lf and/or GOS to increase their functionality, and highly resemble human milk composition (6).
GOS, another functional ingredient, is characterized by its high resistance to digestion and reaches the colon in intact form, where they serve as fermentable substances leading to produce short-chain fatty acids (SCFAs) and decreasing colon pH. These consequences improve the growth of the beneficial bacteria and prevent the attachment of enteropathogens. However, many in vitro, in vivo, and preclinical studies are needed to discover the functionality of human milk components, especially Lf and HMOs. Thus, the main objective of the present study is to evaluate the potential prebiotic efficacy of three different concentrations of recombinant human Lf and/or GOS through using the batch culture fermentation model.
MATERIALS AND METHODS
CHEMICALS
Recombinant human lactoferrin (rhLf) was purchased from Sigma Chemical Co. (cat. no. L1294, St. Louis, MO, United States). Vivinal GOS syrup (dry matter 75 % of which GOS was 59 %, lactose 21 %, glucose 19 %, and galactose 1 %) was provided by Hero Baby Co. (Alcantarilla, Murcia, Spain).
PREPARATION OF THE FECAL INOCULUM
Fecal samples were obtained from three healthy babies (aged 2-4 months) without any known metabolic or gastrointestinal disorders and antibiotics were not taken before fecal sample donation. Fresh fecal samples were immediately placed in anaerobic jars and transported to the laboratory within two hours from collection. Fecal samples were prepared following the procedure previously described by Sánchez-Moya et al. (7).
IN VITRO BATCH CULTURE FERMENTATIONS
Three independent small-scale fecal batch cultures were carried out, each of them corresponding to samples from the three different babies. First of all, 1 % (w/v) lactose was added to the media before autoclaving because it is the main sugar in milk. The fecal slurry and autoclaved MBM were prepared on the same day and maintained overnight under conditions of anaerobiosis at 37 °C, before use. For each batch, MBM was distributed into different glass vessels (5 ml per vessel) with 5, 7.5, or 10 mg of rhLf with (or without) a mixture of 1 % GOS. In addition, 1 % GOS was used as prebiotic control (positive control) (8).
The ingredients assayed (rhLf and GOS) (at the different concentrations) were added to each vessel just before inoculation with the fecal slurry (50 µl of the fecal slurry). The different treatments were incubated at 37 °C in anaerobic conditions. Samples were removed after 0, 10, and 24 h to pH measurement, SCFAs, and bacteria quantification similarly to Beards et al. (8).
The different treatments used in this study are the following: positive control (1 % GOS), single recombinant human lactoferrin (rhLf) (0.10 % rhLf, 0.15 % rhLf, 0.20 % rhLf) and lactoferrin added to 1 % GOS (0.10 % rhLf + 1 % GOS, 0.15 % rhLf + 1 % GOS, 0.20 % rhLf + 1 % GOS).
MEASUREMENT OF pH
The pH values of batch culture samples were measured immediately after taking the aliquots. This measurement was done in triplicate directly by using the pH meter (Crison, Barcelona, Spain).
SHORT-CHAIN FATTY ACIDS ANALYSIS BY GC
SCFAs analysis in fecal cultures was determined by GC according to González-Bermúdez et al. (9). Every sample was performed in quadruplicate.
ANALYSIS OF FECAL MICROBIOTA COMPOSITION BY qPCR
Bacterial DNA from fermented samples was extracted prior to qPCR analysis by using a QIAamp® DNA Stool Minikit (Qiagen, Germany) in a similar form to González-Bermúdez et al. (9). After DNA extraction, samples were stored at -80 °C until further analysis. Quantitative real-time PCR (qPCR) was used to analyze microbiota from fermented samples using specific primers targeting different bacterial groups (total bacteria, Bacteroides, Enterobacteriaceae, Enterococcaceae, Lactobacillus, and Bifidobacterium). PCR amplification and detection were performed similarly to González-Bermúdez et al. (9) and Sánchez-Moya et al. (7) by using a 96-well CFX96™ Real-Time PCR thermocycler (Bio-Rad, Madrid, Spain).
Bacterial strains were obtained from the German Collection of Microorganism and Cell Cultures (DMSZ) and the Spanish Type Culture Collection (CECT) (Table I). Every sample was analyzed in quadruplicate and the results were expressed as the logarithm of genome equivalents per ml (Log genome Eq/ml) (10).
STATISTICAL ANALYSIS
The effect of the different samples at the same in vitro fermentation time, on the evolution of pH, the SCFAs production, and the microbiota growth were tested using one-way ANOVA, with the samples as a factor. To compare the effect of the in vitro fermentation time for each used sample, on the parameters previously commented, one-way ANOVA was used as well, with time as a factor. In both cases, when data were not parametric, the Kruskal-Wallis test was used. Subsequent Tukey’s multiple comparisons or Nemenyi test multiple comparisons were used when data were parametric or not respectively. Before statistical analysis, normality and homoscedasticity were confirmed by using Shapiro-Wilk and Bartlett tests, respectively. The software R version 3.6.2 (2019-12-12) was used to perform all analyses. The package ggplot2 of the same software was used to do plots. A level of significance of p < 0.05 has been considered for each statistical analysis.
RESULTS
It is well-known that there is a positive correlation between gut microbiota and the diet. Thus, the current research is focusing on modulating the gut microbiota using prebiotics such as GOS, and rhLf. The prebiotic substances are several food ingredients that can pass the upper part of the gastrointestinal tract in intact form until reaching the colon, where they are fermented by the colonic beneficial bacteria, especially Bifidobacteria and Lactobacilli. The prominent event that occurs during this process is modulation of the intestinal microbiota composition leading to forming SCFAs as main metabolites, which are accompanied by pH decline.
pH EVOLUTION DURING FERMENTATION
The pH evolution during fermentation for each sample through the time (0, 10, and 24 hours) is shown in table II. As it can be seen, for each sample, pH decreased quickly and significantly (p < 0.05) at ten hours of incubation to the 0-hour point, being this drop bigger for the samples with GOS. At ten hours of incubation, we obtained the lowest value of pH in presence of 1 % GOS (PC), whereas 0.10 % rhLf followed by 0.20 % rhLf and 0.15 % rhLf, respectively, had the maximum values of pH, although without statistically significant differences among the studied samples into ten hours. The values of pH continued decreasing at 24 hours of fermentation as well, with statistically significant differences (p < 0.05) for the 0.1 % and 0.15 % rhLf cases with respect to the previously analysed time, being the lowest value of pH for 1 % GOS. The remaining samples at 24 hours had similar pH mean values and no statistically significant differences were found among the samples used at this time. It is also worthy to note that samples including GOS decrease pH to a higher degree and faster than the same samples without GOS.
SHORT-CHAIN FATTY ACIDS EVOLUTION DURING FERMENTATION
The obtained findings in this study regarding SCFAs production by the fecal bacterial population are shown in figure 1 for the total concentration of SCFA (mM) (acetic, propionic, butyric, i-butyric, i-valeric, n-valeric, i-caproic, n-caproic and heptanoic acids) and in figure 2 for the molar proportions of the main SCFA (acetic, propionic and butyric acid) as well as minor SCFA (i-butyric, i-valeric, n-valeric, i-caproic, n-caproic and heptanoic acid).

Figure 1. Total short-chain fatty acids (SCFA) concentration produced at 0, 10, and 24 hours of incubation with rhLf and/or GOS in batch culture fermentation vessels. GOS: galactooligosaccharides; rhLf: recombinant human lactoferrin.
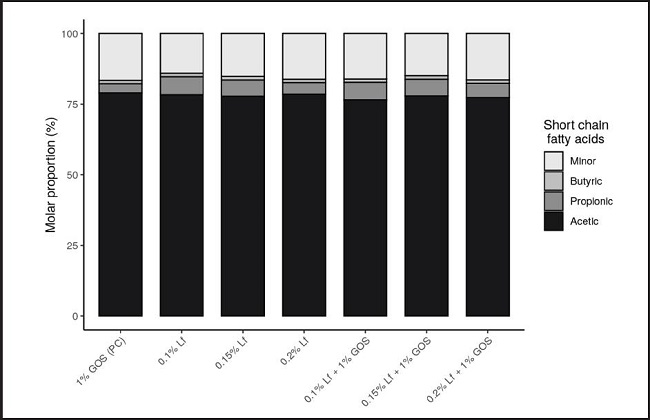
Figure 2. Molar proportion (%) of minor and major short-chain fatty acids (SCFA) concentration produced in batch culture fermentation vessels containing rhLf and/or GOS. GOS: galactooligosaccharides; rhLf: recombinant human lactoferrin.
As it can be seen in figure 1, the total SCFAs levels increased after 24 hours of incubation of fecal microbiota with the tested ingredients. The highest values of total SCFAs were detected after fermentation in the presence of 0.10 % rhLf + 1 % GOS followed by 0.15 % rhLf + 1 % GOS and 0.2 % rhLf + 1 % GOS as compared with the positive control group, but not significantly. The obtained findings revealed, as unexpected, that total SCFAs in the presence of 1 % GOS showed the lowest value after 24 hours as compared to other substrates, revealing the high effect of Lf in the SCFA production. Non-significant differences (p < 0.05) were observed between total SCFAs for all treatments at the same time. Among the various detected SCFAs, acetic acid has been the most contributor to total SCFA, followed by similar amounts of both propionic acid and butyric acid. After 24 hours of fermentation, elevated production of acetic acid was reported, and its higher value was found for 0.10 % rhLf + 1 % GOS followed by 0.20 rhLf + 1 % GOS and 0.15 % rhLf + 1 % GOS as compared with the control group. Unexpectedly, in the presence of 1 % GOS, the acetic acid production was the lowest compared with the remaining samples after 24 hours. It is worthy to note that the highest value of acetic acid after ten hours was reported in presence of 1 % GOS as compared with all treatments. However, non-significant (p < 0.05) changes in the acetic acid production by the tested ingredients were observed at the different points of sampling and this may be a result of the differences between the predominant intestinal flora in the three fecal slurries of the three donors.
In the case of propionic acid, the findings revealed a moderate increase in its level for all the tested ingredients after 24 hours of fermentation. After ten hours of fermentation, the group of samples containing 0.20 % rhLf showed the lowest value as compared with the rest of the treatments, while after 24 hours, the highest value of propionic acid was found in the presence of 0.10 % rhLf + 1 % GOS followed by 0.15 % rhLf + 1 % GOS, and the lowest value was observed in the presence of 1 % GOS. These findings revealed that although there are non-significant (p < 0.05) differences between the values of propionic acid among the different treatments, it might exist a synergistic contribution between rhLf and GOS to produce SCFA. The contrary occurred for butyric acid, which decreased moderately at 24 hours of fermentation as compared to its content at zero time of incubation. The highest value of butyric acid was observed at 24 hours in the presence of 0.20 % rhLf + 1 % GOS followed by the control sample but it is not significantly (p < 0.05) different from the values of the different treatments. Finally, small variability between butyric content of the other groups whether at ten hours or 24 hours was observed.
Interestingly, as a consequence of the SCFAs production pattern mentioned above, the acetic acid/propionic acid ratio highly increased at ten hours of fermentation. Then, this ratio decreased moderately or highly depending on the added compound. Control group acetic/propionic ratio became the highest value at ten hours, while 0.15 % Lf was the lowest value at 24 hours (Fig. 3). Our findings reported that positive control led to a significant change (p < 0.05) in the acetic/propionic acid ratio. Remarkably, the highest decrease in this ratio observed in the present study was for the 0.2 % rhLf group.

Figure 3. Acetic: propionic ratio produced at 0, 10, and 24 hours of incubation with rhLf and/or GOS in batch culture fermentation vessels. GOS: galactooligosaccharides; rhLf: recombinant human lactoferrin.
Because of the obtained findings (Fig. 2), it is worth noting that there was no clear trend related to the effect of rhLf and/or GOS on the production of minor SCFAs in fecal cultures fermentation except for isobutyric acid, which was found to be increased at 24 hours in all treatments as well as in the control group (data not shown).
ANALYSIS OF MICROBIOTA DURING FERMENTATION
Changes in microbiota composition have been shown after the whole fermentation process and using different doses of rhLf and GOS as substrate (Table III). Increments in total bacterial numbers were observed in all samples through the fermentation process, being the time of fermentation ten and 24 hours approximately two Log genome Eq/mL higher with respect to the initial time (time 0 h) (p < 0.05). More counts of total bacteria were observed after using GOS (1 %) and different 0.1 % of rhLf added to GOS (1 %) as fermentable substrate. However, after total fermentation, the addition of different concentrations of single rhLf did not produce significant increases compared to positive control and 0.1 % of rhLf + 1 % GOS.
Table III. Bacterial populations (Log gen Eq/ml) after 24 hours of incubation with rhLf and/or GOS using batch culture fermentation.
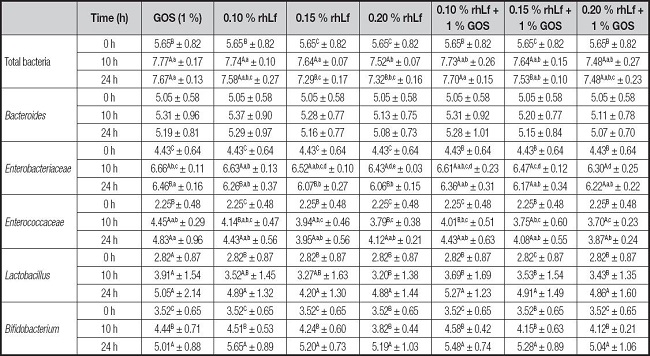
GOS: galactooligosaccharides; rhLf: recombinant human lactoferrin.
Values are mean ± SD of four determinations from three independent experiments.
Small letters denote significant differences (p < 0.05) among different treatments within the same time of fermentation (rows).
Capital letters denote significant differences (p < 0.05) along fermentation within the same treatment.
Considering the same time of fermentation, significant differences were found according to the type of fermented ingredient. Specifically, total bacteria at 24 hours of fermented with 0.10 % rhLf 1 % GOS group and GOS (1 %) were higher compared to 0.15 and 0.20 % rhLf (7.70 ± 0.15 and 7.67 ± 0.13 vs 7.29 ± 0.17 and 7.32 ± 0.16 Log Eq gen/ml, respectively). These data results are interesting since the highest growth of total bacteria in the case of fermented 0.10 rhLf + 1 % GOS was coincident with the highest increases in total SCFA, specifically of acetic acid.
Regarding other bacterial groups, non-significant differences were found in the case of Bacteroides, neither within the same ingredient concerning the time of fermentation nor within the same time of fermentation and ingredient. Unlike other bacteria populations, the growth of Bacteroides group seemed to be decreased from ten to 24 hours of fermentation, although not significantly.
Enterobacteriaceae showed marked time-dependent increases in all samples. Concretely, GOS (1 %), as well as treatments with rhLf alone, showed great differences in the fermentation process. Nevertheless, the addition of 1 % of GOS to rhLf provoked significant increases from time 0 to time ten hours, being the growth after 24 hours of fermentation similar to previous stages. At ten hours of fermentation, the lowest values (p < 0.05) of Enterobacteria were found in the case of 0.20 % rhLf (6.43 ± 0.03) and 0.20 % rhLf + 1 % GOS (6.30 ± 0.25) with respect to the positive control (6.66 ± 0.11). However, in the case of 24 hours of fermentation, the lowest growth for Enterobacteriaceae was detected in the case of 0.15 and 0.20 % rhLf with respect to the control, which could suggest that the addition of rhLf alone to human feces after 24 hours of fermentation produced a positive effect by decreasing this bacterial group. The additive effect of 1 % of GOS and rhLf seemed to produce similar values but none in a significant manner to prebiotic control and a little higher than in the case of 0.15 % and 0.20 % of rhLf.
The lactic acid bacteria, Enterococci, comprises commensal and pathogenic bacteria. The results of the present study showed that all tested ingredients produced increases in Enterococci populations after 24 hours of fermentation regarding the initial inoculum, with increases of 2 Log gen Eq/ml, approximately. However, significant differences were found depending on the ingredient. At the time of ten hours of fermentation 0.10 % rhLf showed similar values than prebiotic control (4.14 ± 0.47 Log gen Eq/ml). Nevertheless, 0.20 % rhLf and 0.20 % rhLf + 1 % GOS showed lesser values with respect to the control. Differently, at the time of 24 hours of fermentation all tested ingredients showed significant similarities with respect to the control, except for 0.20 % rhLf + 1 % GOS, which provoked a diminution of Enterococci growth (3.87 ± 0.24 Log gen Eq/ml).
Regarding Bifidobacterium and Lactobacillus genera, our results showed that both bacteria increased markedly with respect to the initial time over the fermentation, showing a notable prebiotic effect on all tested substrates. Concretely all ingredients stimulated the growth of Lactobacillus, especially after 24 hours of fermentation, showing increments of 2 Log gen Eq/ml approximately with respect to initial values, although insignificant differences were found among different treatments. Similarly, Bifidobacteria showed increases after 24 hours of fermentation using GOS or rhLf as substrate, which could suggest the bifidogenic potential of both ingredients. However, neither of the two mentioned ingredients produced significant increases in Bifidobacterium by comparing different doses.
DISCUSSION
pH EVOLUTION DURING FERMENTATION
One of the most important factors in this topic is pH, which influences the growth and/or activity of intestinal microflora, particularly Bifidobacteria and Lactobacilli. González-Bermúdez et al. (9) indicated that decreasing pH means an increase in acidity due to increased production of SCFAs by the fecal microbiota, and reported a negative significant relationship between pH and acetic acid or total SCFAs in all sampling points.
The decreasing tendency in pH found in our study could be explained because antimicrobial properties of Lf have been reported, which reduce bacteria growth (11); meanwhile, GOS induced a fast fermentation, softening the Lf effect. At 24 hours of fermentation, pH values were similar in all samples, maybe because Lf has been degraded and its antibacterial properties have been erased.
Recently, the drop in pH from the ileum to the cecum due to the higher SCFA concentrations has had two beneficial effects. First, both in vitro and animal studies showed that lower pH values change gut microbiota composition, and secondly, it prevents overgrowth by pH-sensitive pathogenic bacteria like Enterobacteriacae and Clostridia (12). Regarding the role of GOS as a fermentable substrate, it has been demonstrated that it produced a large concentration of lactate after 24 hours of fermentation, reducing pH values (13), which could explain the considerable decline in pH values in this study.
SHORT-CHAIN FATTY ACIDS EVOLUTION DURING FERMENTATION
SCFAs are organic acids with 1-6 carbon atoms and are one of the main anions obtained by the degradation of polysaccharides, oligosaccharides, proteins, peptides, and glycoprotein precursors in the colon by gut microbiota. Currently, it is well confirmed that produced SCFAs have several health-promoting activities such as improving water transport, sodium absorption, and bicarbonate excretion (14). Furthermore, SCFAs play a pivotal role as nutrients for the colonic epithelium, modulators of colonic and intracellular pH, and other functions related to Fe transport, and regulators of proliferation, differentiation, and gene expression. The accumulated SCFAs decrease the colonic pH leading to modulation of the pattern of colonic microbiota, and also improve the Ca and Fe absorption (15). SCFAs accumulated in the colon act as lowering factors of bioavailability of the toxic amines, thus protecting from the carcinogenic progress.
The SCFA evolution in our study was in line with the obtained findings by Miller and Wolin (16), revealing that acetic acid was the major SCFA accumulated after fermentation of glucose purified from cabbage. Also, it was reported by Velazquez et al. (17) that glucose fermentation by intestinal microbiota gave rise to more acetic acid but less propionic and butyric acid production than other fermentable substrates. In addition, acetic acid and total SCFAs were highly produced after short-term incubation of lactulose and GOS with intestinal flora. Likewise, Beards et al. (8) reported that the colonic fermentation of GOS induced the production of acetic acid, which correlated positively with the increase of Bifidobacteria populations, as acetate formation is consistent with Bifidobacteria and Lactobacilli metabolism. Similar results have been reported showing that the increasing production of acetic acid was directly associated with the increase in Bifidobacteria counts (18). Several published data revealed that prebiotics are the main responsible for the marked increase in the counts of Bifidobacteria, Lactobacilli and, subsequently, the higher production of acetic acid and total SCFAs (19) , and these changes may be responsible for promoting the defense functions of the host and thereby protecting the host from severe infection (20).
It was previously reported that propionate may form a soluble complex with iron, thereby maintaining the solubility of iron in the lumen of the colon, as well as facilitating the transfer across endosome membranes of the enterocytes (15). The low concentration of butyric acid found in our study after 24 hours of fermentation has been related to reductions of Clostridium and Enterobacteriaceae numbers. The main butyrate-producing bacterial groups found in human feces, as well as related to the decrease of Faecalibacterium prausnitzii (Fpra655) populations has been reported in a recent study (21) . However, the present findings showed increases of Enterobacteriaceae for 24 hours of fermentation. Furthermore, butyric acid production was not observed in these mostly acidic cultures when pH values of fecal cultures fall below 6.0 as indicated to occur by Walker et al. (22). Likewise, many studies revealed that acetic acid is produced at higher levels than propionic and butyric acids (23). Rossi et al. (24) indicated that the presence of FOS and inulin highly affected the production of SCFAs. Fermentation of FOS led to producing acetic and lactic acids as the main fermentation end products, while fermentation of inulin rose butyric acid level. Thus, because of the previously reported data, we can report that the type of dietary components, their composition, polymerization degree and intestinal microbiota pattern of infants, and the age of the infant may greatly influence on the accumulated SCFAs.
Respecting the acetic acid/propionic acid ratio, Delzenne and Kok (25) reported that its decreased could be suggested as a possible marker of the hypolipidemic effect of prebiotics (as noted by the inhibition of cholesterol and fatty acids biosynthesis in the liver, which finally results in a decrease in lipid levels in the blood).
It is well-known that the feeding pattern of the infant could influence the predominant microflora (type, composition, and activity) in the infant’s gastrointestinal tract. The diet is the main factor in modulating the gut microbiota. This effect is greatly observed in the intestinal microflora pattern predominant in the infant gut, where Bifidobacteria and Lactobacilli are predominant in breast-fed infants gut (5), and breast human milk contains several components with a prebiotic nature, such as HMOs. The fermentation of this prebiotic can stimulate the selective growth of Lactobacillus and Bifidobacteria species and the subsequent production of SCFAs. Many published studies have shown that acetic acid was the main fermentation end-product in infants that received human milk while propionic and butyric acids were the predominance metabolites in the gut of bottle-fed infants. Various studies confirmed that the beneficial effect of prebiotics added to infant formulas strongly influenced SCFAs pattern providing a fecal SCFAs profile that is close to that of breast-fed infants, with a high level of acetate and lower level of propionate and butyrate (26).
In short, SCFAs (acetate, propionate, or butyrate), the main end-products resulting from microbial fermentation of these indigestible oligosaccharides, are well-known to possess beneficial effects on the host. Enhanced SCFAs production and increased delivery of these compounds in the distal colon, especially butyrate, may have a role in preventing colon cancer and other intestinal disorders (27). Furthermore, these metabolites can improve mineral absorption by their role in decreasing the pH of colon contents (15) leading to enhancement of the released iron from its complex with proteins, thereby increasing its bioavailability (15). Also, SCFAs possess beneficial effects on the intestinal level, as they may stimulate the proliferation of epithelial cells, thereby increasing the absorptive surface area in the colon. Likewise, prebiotics or their metabolites may create an environment in the colon that promotes the reduction of Fe3+ to Fe2+ (28) . It may stimulate the expression of iron regulatory genes, thereby increasing iron absorption, such as calbindin-D9K, which positively affect Ca absorption and DMT1, hemochromatosis protein (HFE), duodenal cytochrome b (Dcytb), and ferroprotein-1 which positively influenced Fe absorption (29).
ANALYSIS OF MICROBIOTA DURING FERMENTATION
Both ingredients, lactoferrin and GOS, could serve as a substrate for microbiota, giving place to prebiotic effects (8). Different concentrations of rhLf did not produce significant increases in total bacteria, what could be explained because of the effect of GOS. In this sense, Rycroft et al. (13) showed little insignificant increases in the total count after the use of GOS after five and 24 hours of batch culture fermentations. Nevertheless, other researchers showed significant increases in total bacteria after 24 hours of batch culture fermentation using GOS as substrate (8).
Regarding Bacteroides, an increase in this bacterial group has been related to beneficial effects on gut health, contributing to the synthesis of different vitamins and reducing gut inflammation (30). However, our results showed a decrease in this group from 10 to 24 hours of fermentation, which are in agreement with other authors that demonstrated that Bacteroides decreased after fermentation (7) due to the fall of pH (5.5 or lower) (31). In addition, Bacteroides could produce propionate using lactate as substrate, which could explain the poor production of propionic acid shown in our results (31).
As it has been previously mentioned in the case of total bacteria, it could exist a relationship between increases in Enterobacteria, SCFA production, and the addition of GOS to rhLf. At this point, it is important to note that this heterogeneous family of bacteria comprises a wide number of members of microorganisms, which can remain as a commensal organism or produce enteric diseases and dysbiosis (32). Therefore, the present results would indicate increases in desirable bacterial groups through fermentation of GOS and rhLf. However, the antimicrobial activity of lactoferrin has been widely proved. Concretely, bacteriostatic and bactericide functions have been attributed to lactoferrin, which has great implications for human health (2). These effects against a great number of G+ and G- bacteria could be mediated through the alteration of membrane permeability of bacteria or/and by sequestering the iron needed for bacteria nutrition. Several studies have demonstrated the inhibitory effect of lactoferrin against pathogens such as Escherichia coli and Listeria monocytogenes (33). Nevertheless, the present results suggest that single lactoferrin or GOS added to lactoferrin at different concentrations did not inhibit the growth of Enterobacteria, maybe because a higher amount of lactoferrin could be necessary to achieve the bacteriostatic/bactericide effect. In this sense, the oral administration of bovine lactoferrin in obese mice modestly inhibited the growth of these groups of bacteria (34). Regarding the effect of prebiotics, such as GOS, on Enterobacteria’s growth culture fermentation had proved that GOS stimulated the growth of E. coli after four hours of fermentation, although this increase did not continue over time (eight and 12 hours) (35). In vivo studies showed that the administration of several dosages of GOS to human volunteers did not change the levels of lactose-fermenting enterobacteria on fecal samples (36). These contradictory results could be due to differences in the experimental design, time of incubation, prebiotic dose, and the complexity of the gut environment.
Some species of Enterococci are recognized as pathogens although other members are related to antimicrobial activity because of the production of bacteriocins. In this sense, some members of the genus Enterococcus, such as E. faecium or E. faecalis, have been studied because of their probiotic potential. This probiotic effect has been demonstrated in the prevention and/or treatment of irritable bowel syndrome and chronic intestinal diseases (37). Our results could suggest that GOS and rhLf stimulated the growth of Enterococci over the fermentation. In line with our results, both GOS and lactoferrin have shown stimulants effects on the growth of Enterococcaceae. However, other researchers did not show any dose-effect when GOS was administered to human volunteers (36).
Regarding Bifidobacterium and Lactobacillus genera, our results showed an increase in both groups along fermentation. A wide number of studies have proved the prebiotic effect of both GOS and lactoferrin. Specifically, Rycroft et al. (13) found increases in the number of Bifidobacteria after five and 24 hours of fermentation and lesser effect in other groups of bacteria. Li et al. (38) demonstrated that GOS stimulated selectively the growth of Bifidobacteria after 24 hours of fermentation using fresh human feces. Similarly, other authors demonstrated the growth of Lactobacilli and Bifidobacteria after a batch culture fermentation using GOS as substrate (35). Many researches have proved the growth-promoting effect of lactoferrin on Bifidobacteria. The bifidogenic effect could be mediated by peptides derived from lactoferrin, bound iron, and sugar chains (4). For instance, in vivo studies have shown that the oral supplementation of bovine lactoferrin to obese mice increased the number of Bifidobacterium spp. after 12 weeks of treatment, which suggested the prebiotic potency of this protein (34). In addition, lactoferrin appears to modulate gut microbiota, favoring the growth of Lactobacilli and Bifidobacteria and decreasing the counts of pathogenic bacteria (39).
CONCLUSION
Despite the importance of the batch culture fermentation technique in uncovering the prebiotic activity of the food ingredients, it is not useful for detecting the prebiotic nature of Lf due to its nature as a protein. Thus, Lf maybe shows its prebiotic activity on the gut microbiota through other mechanisms.