There is increasing interest in the study of environmental factors that may affect human neurodevelopment in industrialised countries. In particular, the impact of exposure to early life stress (ELS), together with the consumption of diets that are high in saturated fats and processed carbohydrates such as sugars (also known as the Western diet pattern) is still a relatively unexplored research topic that represents a serious public health concern (López-Taboada et al., 2020; Tsan et al., 2021). Even considering that the aetiology of many mental disorders is multifactorial and remains currently unknown, it has been acknowledged that diet and exposure to ELS can contribute to the risk of developing a wide variety of mental health problems (Morris et al., 2015; Rincel et al., 2016)
Traumatic experiences and early adversity during childhood have been widely linked to an increased risk of developing depression or anxiety disorders in adulthood (Chen et al., 2010; Nemeroff, 2016). The association between child abuse, neglect, and depressive or anxiety disorders has been extensively studied. ELS is associated with cognitive impairment, altered stress, and emotional responses in both humans and animal models (Cao et al., 2016; Rincel et al., 2019).
Other key environmental factors of interest include nutrition and diet. Specifically, the western diet is the most ubiquitous environmental factor associated with obesity, overweight, and non-communicable diseases (López-Taboada et al., 2020). This diet is characterized by being hypercaloric, highly palatable foods, and is mainly composed of saturated fats, simple sugars, and salt (Francis & Stevenson, 2013). More precisely, consuming a western diet has been reported to impair spatial learning and hippocampal neurogenesis, increase oxidative stress, and promote neuroinflammatory processes (Sasaki et al., 2013; Tsan et al., 2021) among other impacts on neurodevelopment and behaviour.
In contrast, the colon is the most populated region in pools of commensal microorganisms (microbiota), including bacteria, archaea, viruses, and unicellular eukaryotes (Salazar et al., 2019). It has been demonstrated that there is a bidirectional connection between the brain and gut microbiota called the gut-brain axis (Cryan et al., 2019). The gut-brain axis involves the enteric nervous system (ENS), autonomic nervous system (ANS), central nervous system (CNS), and hypothalamic-pituitary-adrenal (HPA) axis (López-Taboada et al., 2020). Therefore, gut microbiota influences behaviour and CNS function through several direct and indirect mechanisms (Cryan et al., 2019). Many rodent and human studies have shown that gut microbiota affects host metabolism (Karl et al., 2018; Wang et al., 2018). A wide range of environmental factors like dietary patterns, anxiety-like behaviour, and depression-like behaviour may modify diversity and concentration of gut microbiota and influence the gut-brain axis (López-Taboada et al., 2020).
Different studies have reported that ELS can program the development of the HPA axis stress response, and the consumption of a western diet could be a cumulative adverse environmental factor (cumulative stress hypothesis) that increases adult stress responses and the risk of overweight, obesity, and mental disorders (Agorastos et al., 2019). However, other studies suggest that consumption of a western diet could also be considered a coping mechanism in response to ELS, as it is related to the modulation of the development of the HPA axis stress response by palatable foods, which may counteract the adverse effects of ELS on the adult stress response (Rincel et al., 2016; Tsan et al., 2021). Moreover, some authors have suggested that consumption of high-fat and high-sugar diets during pregnancy and lactation could not only partially counteract the negative effects of ELS by maternal separation on adult anxiety-like and depression-like behaviours but also revert gut leakiness or increased gut permeability caused by ELS (Rincel et al., 2018). In this context, the main objective of this study was to evaluate the effect of an exposure to a high-fat and high-sugar (HFS) diet and early-life stress (ELS) on adult male Wistar rats, each factor independently and after a combined exposure, to assess the gut microbiota composition, anxiety- and depression-like behaviours. We hypothesised that, in accordance with the abovementioned studies, the interaction between maternal and postnatal exposure to an HFS diet and ELS by maternal separation would modify the effects on adult emotional behaviour and microbiota composition caused by independent exposure to these adverse environmental factors.
Method
Participants
In order to carry out this research, 10 adult female Wistar rats from the vivarium of the University of Seville (Spain) were used. Animals were bred in the central vivarium of the University of Oviedo and housed with two adult male Wistar rats, from the vivarium of the University of Seville, in transparent plastic boxes (45 × 24 × 21 cm) for at least 24 h. Once this was completed, each litter was randomly assigned to the experimental treatments (diet and exposure to maternal separation) using n =40 male Wistar rats altogether, n =10 per group. Animals were kept in the vivarium at a controlled temperature of 21± 2 °C and 60± 5% relative humidity, following a 12:12 h light/dark schedule with lights turned on at 07:00 h daily. The animals were provided food and drink ad libitum. All experimental procedures were performed following the European Union Directive 2010/63/UE of September 22, 2010, and were approved by the Ethical Committee in Research of the University of Oviedo (Government of the Principality of Asturias, Consejería de Desarrollo Rural y Recursos Naturales, Spain) on December 4th, 2018 (PROAE 35/2018). The investigators were blinded to the experimental groups, including behavioural tests and microbiota analysis.
Instruments
Maternal Separation Procedure
A widely used maternal separation protocol was followed (Lippmann et al., 2007) starting on postnatal day 2 and concluding on postnatal day 14. The instrument used to place the offspring separated from their mothers was an incubator (Cosmo EVO 72, FIEM SRL, Como, Italy) that provided adequate temperature and humidity conditions (60% humidity and 30 °C temperature).
Diet Requirements and Experimental Groups
One of the diets chosen for half of the dams and their offspring was a standard maintenance diet, low in saturated fat and without free sugars (SD) (Diet TD.06415, Envigo Teklad Diets, Barcelona, Spain), which was composed of 13% kcal vegetal fat (from soybean oil), 20% kcal protein, and 67% kcal carbohydrates (from wheat and corn) for a total of 2900 kcal/kg. In contrast, the other half of the dams and their offspring received a hypercaloric diet high in fat and sugars (HFS) (obesogenic Diet D12451, Research Diets, New Brunswick, NJ, USA) composed of 45% kcal saturated fat (from lard), 20% kcal protein, and 35% kcal carbohydrates, reaching a total of 4057 kcal/kg, with 17% kcal sucrose, representing 20% of the total weight.
Elevated Zero-Maze Test
The elevated zero maze consisted of an elevated structure with a circular shape and black colour. This structure is divided into four quadrants, two of which are uncovered and two of which are covered by walls of approximately 20 cm in height, all of which have the same size. Owing to the properties of the maze, it is ideal to generate a conflict between the tendency to explore and the need to shelter rodents from possible dangers. Thus, this test was used to evaluate anxiety-like behaviour in rats (Shepherd et al., 1994).
The automated behaviour analysis system Ethovision XT (Noldus Information Technology, Wageningen, The Netherlands) records multiple variables, namely, time spent in open or closed sectors, total distance moved, and frequency of visits to open or closed sectors.
Forced-Swim Test
The forced swimming test allows the assessment of depression-type behaviour, originally described as "behavioural despair" ̶ test in rodents by Porsolt (Porsolt et al., 1978). To perform this test, a cylindrical translucent glass (height:50 cm, diameter:25 cm) covering up to 35 cm with tap water at a temperature of 25 ± 2°C was required. This test is widely used to evaluate the antidepressant effects of drugs in rodents by measuring total immobility time in a water-filled cylinder. Animal movements were tracked using an automated video monitoring and behavioural analysis system (Noldus Ethovision XT), which collects variables such as swim speed, total distance swum or the percentage of time the rat was immobile, which is the most informative variable.
Saccharin Preference Test
Anhedonia-related behaviour was evaluated using a saccharin preference test (Rabasa et al., 2016) in 57-day old rats. Individual cages were used with free access to two different bottles for 24 h, one with saccharin solution (0.1% w/v) and the other with tap water. The consumption ratio was calculated using the following formula: saccharin intake / (saccharin intake (g) + water intake (g)) × 100).
Faecal Pellets Processing and Analysis of Microbiota
Faecal pellets were homogenised in PBS buffer (1:7 w/v) for 3 min at full speed using a stomacher (LabBlender 400; VWR, Radnor, PA, USA). Cell pellets and faecal supernatants were separated by centrifugation (10.000 rpm, 15 min) and stored at -20 °C for further analyses.
Cell pellets were used for bacterial DNA isolation using the QIAamp DNA stool kit (Qiagen, GmbH, Germany), following the manufacturer’s instructions. The isolated DNA was used as template for partial sequencing (hypervariable V3-V4 region) of the 16S rRNA gene on the Illumina platform using the pair primers 341F (CCTAYGGGRBGCASCAG) and 806R (GGACTACNNGGGTATCTAAT) following in-house protocols (Novogene, Beijing, China). Based on the unique barcodes of the samples, raw paired-end reads were assigned to different samples and merged into raw tags using FLASH (version 1.2.7) (Magoč & Salzberg, 2011). The merged raw tags were filtered and developed into clean tags using the QIIME (version 1.7.0) quality-controlled process (Bokulich et al., 2013; Caporaso et al., 2010). Clean tags were then compared with the reference Gold database (Release 20110519), and chimera sequences were detected and deleted using the UCHIME Algorithm (Version 7.0.1001) (Haas et al., 2011). The non-chimera clean effective tags were clustered into OTUs with ≥ 97% similarity using Uparse software (Version 7.0.1001) (Edgar, 2013). The representative sequence for each OTU was selected, and taxonomic information was annotated using the SILVA database (Quast et al., 2013).
The faecal supernatant was used for short-chain fatty acid level determination using gas chromatography, as previously described (Moris et al., 2018). Briefly, the supernatant was mixed with a solution of methanol, formic acid, and an internal standard, centrifuged, and injected into a gas chromatograph (GC) composed of a 6890N GC injection module (Agilent Technologies Inc., Palo Alto, CA, USA) connected to a flame injection detector and a mass spectrometry 5973N detector (Agilent).
Procedure
Maternal Separation Procedure
The procedure started on postnatal day 2 and concluding on postnatal day 14, separating the offspring from the separation group (MS) daily for 180 min and placing them in the incubator. Simultaneously, the early handling (HD) control group underwent brief maternal separation daily for 15 min, while each litter was handled by the experimenters. On day 21, the pups were separated from their mothers and placed in boxes, each consisting of a group of rats, as described later. Rat body weight gain was measured twice per week from day 1 to day 60.
Diet Requirements and Experimental Groups
Four different groups were established for the dams whose offspring were used according to the consumed diet (HFS or SD) and exposure to maternal separation after birth (HD or MS): SD-HD: Standard Diet and Handling. HFS-MS: High-fat and high-sugar diet and Maternal Separation. SD-MS: Standard Diet and Maternal Separation. HFS- HD: High-fat and high-sugar diet and Handling. Therefore, two groups received the HFS while the other two received the SD both starting with their respective diets at least 3 weeks before mating (dams), during the gestation period, and postnatally until adulthood (60 days). Male rats (n =10 per group) were behaviourally tested at 55 days of age, and faecal samples were obtained from each animal after completion of the behavioural tests (see Figure 1 for the experimental timeline). In summary, 40 rats were used in the present study.
Elevated Zero-Maze Test
The procedure consisted of introducing the animals into the maze for 5 min. Specifically, the rats were placed in front of one of the covered arms and allowed to explore the entire maze for 5 min on PND 55. Then, the automated behaviour analysis was used to record relevant variables as the time spent in open or closed sectors or total distance moved.
Forced-Swim Test
The test was performed on 2 consecutive days in 55-day-old rats. On the first day, the animals were placed in water for approximately 15 min, allowing them to swim and explore freely. The next day, this procedure was repeated but for 5 minutes only. Meanwhile, the automated tracking system collected variables such as swim speed, total distance swum or the percentage of time the rat was immobile.
Saccharin Preference Test
On the following day, anhedonia-related behaviour was evaluated using the saccharin preference test in 57-day old rats. Animals were housed in individual cages with free access to the two different bottles for 24 h. The bottles were weighed before and after the assay and the rats were weighed. We did not apply any prior food or water deprivation before testing.
Analysis of Faecal Microbita
Fresh faecal samples were collected by massaging 60-days-old male rats from each experimental group. Faecal samples were packed immediately in small airtight plastic bags and stored at -80 °C. Later in the laboratory, the faecal samples were analysed by 16S rRNA Gene Profiling and, subsequently, short-chain and branched-chain fatty acids were measured following the protocol and using the instruments described before.
Data Analysis
Differences in body weight between the experimental groups were analysed using two-way repeated measures analysis of variance (ANOVA) with treatment (SD-HD, SD-MS, HFS-HD, HFS-MS) and age as repeated measures. Holm-Sidak method was used for post-hoc analyses.
Data from the behavioural tests were analysed using a two-factor ANOVA, with diet (SD vs. HFS) and rearing (HD vs. MS) as independent variables. Post-hoc analyses were performed using the Holm-Sidak method when interactions between variables were significant. All analyses were performed using SigmaPlot 12.5 (Systat Software Inc., Richmond, CA, USA) software with the level of significance set at p ≤ 0.05.
Data from the gut microbiota were log2 transformed to account for the non-normal distribution of taxonomic count data, TSS, and CSS normalised (Paulson et al., 2013), and analysed using two-way ANOVA, with diet and rearing as independent variables. Post hoc pairwise analyses were performed using Tukey’s test. Alpha diversity indices (Chao1 and Shannon) were also calculated and analysed by two-way analysis of variance (ANOVA) using normalised data. Principal component analysis (PCA) and PERMANOVA tests were performed to analyse beta diversity. The LefSe test (linear discriminant analysis effect size) was used to detect species features between groups. Hierarchical clustering was performed using Pearson’s correlation as a distance metric from the relative abundances at the genus level. Data from SCFAs were analysed using two-way analysis of variance (ANOVA), with diet and rearing as independent variables. Statistical analyses were computed using the following free software: R v3.2.5, Calypso, and Multi-Experiment Viewer software (MEV). Data were considered statistically significant at p < 0.05.
Results
Body Weight
Figure 2 shows the mean body weights of all experimental groups measured twice a week for 60 days. Specifically, two-way repeated measures ANOVA indicated significant main effects of treatment (F(3,504) = 89.9, p < .001), age (F(14,504) = 2516.52, p < .001), and interaction between treatment and age (F(42,504) = 34.08, p < .001). Post-hoc analysis of the interaction showed that the HFS diet groups (HFS-HD and HFS-MS) had significantly higher body weights than the SD (SD-HD and SD-MS) groups (p < .001) from postnatal day 28.
Elevated Zero-Maze
To evaluate differences in anxiety-related behaviour between the experimental groups, three different variables were measured: time spent in the open arms, number of entries into the closed arms, and distance moved. Two-way ANOVAs revealed a significant interaction between diet and ELS in the percentage of time spent in the open arms (F(1,39) = 14.10, p < .001) and the number of closed sector entries (F(1,39) = 7.06, p ≤ 0.01). The post-hoc analysis showed that HFS-HD animals spent significantly less time in the open arms than both the SD-HD (p < .001) and HFS-MS (p ≤ 0.01) groups (Figure 3a). Furthermore, the analysis showed that exposure to maternal separation decreased the percentage of time spent in the open arms in the standard diet group (p < 0.01) SD-HD versus SD-MS but increased it in the HFS-MS group versus the HFS-HD group (p < .001). Similarly, there was a significant decrease in the number of entries into the closed arms in the HFS-HD animals compared to the SD-HD (p < .001) and HFS-MS (p < 0.05) groups (Fig. 3b). No differences between the groups were found in the distance travelled (Figure 3c).
Forced-Swim Test (FST)
Two-way ANOVA analysis of the total duration of immobility in the FST showed an interaction effect between diet and early life stress by MS (F(1,39) = 19.27, p < .001; Figure 4) SD-HD and HFS-MS groups spent more time immobile than the animals of the SD-MS (p ≤ 0.01) and HFS-HD groups (p < .001).
Saccharin Preference Test
Analysis of the percentage of saccharin solution consumed relative to total fluid consumption using a two-way ANOVA demonstrated an effect of diet (F(1,39) = 6.99, p ≤ 0.01), showing that the SD groups had a significantly increased preference for saccharin compared to the HFS diet groups (p ≤ 0.01) without any significant differences due to MS (Figure 5).
Microbiota Diversity Assessment
Analyses of the 16S rRNA gene profiling of faecal samples showed differences in the faecal microbiota composition between the four groups of study, as evidenced by their clear separation in the principal components analysis (PCA) (p < .001) (Figure 6a). The first component, explaining 39% of the variance, established a clear separation depending on the diet, and the second component, explaining 12% of the variance, grouped closer to the SD-HD and HFS-MS rats. Evaluation of the Chao 1 richness estimator did not reveal any significant differences between the groups when the interaction between the two variables (diet and ELS) was studied (Figure 6b). However, the Shannon index revealed a statistically significant lower diversity in the HFS-HD group (p <0.05) and a trend toward lower levels in the SD -MS group (p = .05) than in the SD-HD group (Figure 6b).
Microbiota Composition
Firmicutes was the most abundant phylum, followed by Bacteroidetes, reaching between those two phyla a relative proportion of 95% of the faecal microbiota in all the experimental groups (Figure 7). Changes in the gut microbiota due to both factors studied (diet and ELS) were assessed at the phylum level.
Although a significant effect was found for ELS (p < 0.01) in the independent tests, showing lower abundances of Firmicutes in both ELS-groups regarding the HFS-HD (p < 0.01) and lower relative proportions of Proteobacteria in the SD-MS group with respect to handled rats, when the two study factors were studied together, no statistical differences were observed. Bacteroidetes, which seemed to be more affected by diet (p < .001) than by ELS (p = 0.590) when both factors were studied independently, was significantly higher in the SD-HD group with respect to both HFS diet groups (p < .000 versus the HFS-HD group; p < 0.05, versus the HFS-MS group) when focusing on the interaction of both factors. Furthermore, some changes were observed as a result of the interaction between diet and ELS in minority phyla. Saccharibacteria were significantly lower in the HFS-MS group with respect the others three (p < 0.01). Conversely, the HFS-MS group showed significantly higher relative proportions of Deferribactereaceae than HFS-MS and SD-MS groups (p < 0.01).
At lower taxonomic levels (Figure 8) the family Lachnospiraceae was the most abundant in all groups, except in SD-HD rats where the Bacteroideales_S247 group was dominant. Ruminococcaceae, Prevotellaceae, and Lactobacillaceae were the next most abundant families (Figure 8). Although a greater number of families belonging to the Firmicutes phylum was affected by the diet, a larger number of Bacteroidetes families showed significant changes when the interaction of both factors was considered. Among them, the SD-HD group showed significantly (p < 0.0001) higher relative abundances of the Bacteroidales _S247 family than the HFS diet group, and HFS-HD displayed lower proportions of the Bacteroidales _S247 family (p < 0.01) than the SD-MS group. Prevotellaceae is affected by both factors (p < 0.0001 diet; p < 0.0001 ELS), being lower in HFS- HD group with respect to the other three groups (p < 0.0001). Rikenellaceae was also higher (p < 0.01) in SD-HD with respect to SD-MS and HFS-HD. Interestingly, at the genus level, Bacteroides increased (p < 0.05) in the HFS-MS group with respect to HFS-HD and SD-MS, with similar relative abundances to SD-HD. No significant changes were observed in Lachnospiraceae and Ruminococcaceae among the four groups of rats. On the other hand, the levels of Lactobacillaceae and Erysipelotrichaceae were significantly lower in the SD-HD (p < .001) and HFS-MS (p < 0.05) rats with respect SD-MS and HFS- HD, but not between the two of them neither between SD-MS and HFS- HD groups. At the genus level, these observations were confirmed by Lactobacillus abundance (p < 0.0001), which was also higher in the HFS-HD group than in the SD-HD group (p < 0.0001). For Proteobacteria, different families were significantly affected (p < 0.05) by ELS and diet, but the interaction of both factors led to lower relative abundances of Desulfovibrionaceae in SD-MS (p < .001) and Rhodospirillaceae in HFS-HD (p < 0.05) than in the other three groups, lower proportions of Rhodospirillaceae in SD-MS than in SD-HD, and higher Deferribactereaceae abundance in HFS-MS (p < .001). Although Verrucomicrobiaceae were affected by diet (p < 0.01), the interaction with ELS did not exert any significant change in its relative abundance.
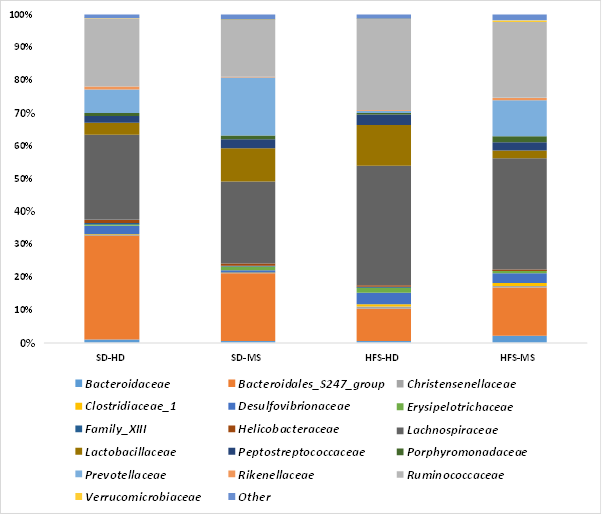
Figure 8. Relative Percent Abundance of Different Bacterial Families in the Faecal Samples of the Four Experimental Groups.
With the objective of identifying phylotypes responsible for the differences observed among groups, a linear discriminant analysis effect size (LEfSe) analysis among groups was carried out. When the four groups were compared, Firmicutes families had the greatest discriminatory power for the HFS-HD group. Among them, Lachnospiraceae, Ruminococcaceae and Lactobacillaceae showed the highest LDA scores. Conversely, Bacteroidetes families were found to best characterise the microbiota profile of the other three experimental groups. When pairwise were done and the control SD-HD group as compared against HFS- HD and HFS-MS groups independently, Bacteroidales_S4_7 at family level was also discriminative of control group; and Lachnospiraceae, Ruminococcaceae were most associated to groups fed the HFS diet. However, LEfSe analysis identified Desulfovibrionaceae as the discriminating taxon for the SD-HD control group, whereas Lactobacillaceae was discriminative for the SD-MS group based on the gut microbiota of the standard diet groups. Moreover, hierarchical clustering (HCL) at the genus level showed that diet was the main cluster variable; however, interestingly, the microbiota composition from the HFS-MS group clustered closer to that of the SD groups than that of the HFS-HD group.
Microbiota Metabolism
Acetate had the highest concentration of SCFAs, followed by butyrate and propionate (Figure 9). The concentrations of the three SCFAs were affected by the ELS (p < 0.05) and increased in the MS groups when compared with the HD animals in both diet groups. No statistically significant interaction was observed between the ELS and diet.
Discussion
In agreement with our results, the combined exposure to the HFS diet and ELS reversed some of the effects on behaviour and microbiota composition compared to independent exposure to only one of these environmental factors. Accordingly, we found that combined exposure to an ELS and HFS diet partially reversed abnormal anxiety-like and depression-like behaviours induced by an ELS or HFS diet independently, and the results obtained were comparable to those of the control group.
Regarding behavioural outcomes, prolonged exposure to an HFS diet (prenatally and until early adulthood) in the control group increased anxiety-like behaviour in the elevated zero-maze, but decreased immobility in the forced swim test and decreased preference for saccharin, a test for anhedonia, compared to all other groups. Maternal hypercaloric HFD consumption has been reported to increase anxiety- and depression-like behaviours in the offspring of rats and mice (Fernandes et al., 2021; Johnson et al., 2017;Winther et al., 2018). Moreover, most studies have reported that postnatal exposure to obesogenic diets high in fat and sugar increases anxiety- and depression-like behaviours in rats and mice (Aslani et al., 2015; Yang et al., 2020). Conversely, some studies have suggested that the consumption of hypercaloric or obesogenic diets specifically decreases anxiety-like behaviours in rodents (Maniam & Morris, 2010; Sasaki et al., 2014). These contrasting results about the effects of obesogenic diets on anxiety-like behaviour could be related with the type of diet used, since ‘cafeteria’ diets containing highly palatable food (like chocolate, biscuits, cheese, etc.) could be associated with anxiolytic effects (Pini et al., 2017) due to the rewarding effects of highly palatable foods and the exposure to novel or diverse foods. In addition, it has recently been reported that the content of saturated fatty acids (from lard, such as the diet used here) is particularly associated with increased anxiety-like behaviour in high-fat diets (Souto et al., 2020).
However, the control group fed the HFS diet showed decreased immobility in the FST compared to all other groups, which could be interpreted as an antidepressant effect of the HFS diet. In this regard, increased immobility in the FST has traditionally been considered behavioural despair or depression-like behaviour in rodents (Porsolt et al., 1978). The results showed that rats in the control (brief handling) group fed the HFS diet (HFS-HD) showed decreased immobility in the FST. Increased locomotor activity in the FST can also be interpreted as increased anxiety-like behaviour (Anyan & Amir, 2018) or impaired stress-coping ability (Armario, 2021; Commons et al., 2017). However, the decreased relative saccharin preference in this group could be interpreted as decreased motivation for rewarding stimuli or anhedonia, a key characteristic of depression (Scheggi et al., 2018).
Moreover, HFS-HD rats showed a significantly decreased proportion of the Bacteroidetes phylum compared to the briefly handled group fed the standard diet; consequently, an increased proportion of the Firmicutes phylum was observed in this group. This result is in line with previous studies on the effects of high-fat diets on the gut microbiota of rats and mice (Johnson et al., 2017). In particular, we found a decreased abundance of some Bacteroidetes families, such as Bacteroidales _S24-7, Prevotellaceae, and Rickenellaceae, in the HFS-HD group, which is consistent with other studies (Malesza et al., 2021). Changes in Firmicutes families were also observed, with commonly observed higher levels of the Lactobacillus genus (Malesza et al., 2021) after HFD. These changes in the composition of the gut microbiota induced by the HFS diet have been associated with increased gut permeability, inflammation, and anxiety-like behaviour in mice (Oriach et al., 2016). Since HFS-HD rats showed anxiety-like behaviour and anhedonia compared to all other groups, microbiota alterations induced by the HFS diet could be involved in the behavioural results reported here.
On the other hand, exposure to early life stress by MS in rats fed a standard diet (SD-MS) increased anxiety-like behaviour in the elevated zero-maze as compared to the early handled rats fed the same diet. This result supports previous studies that reported increased anxiety-like behaviour in adult rats that underwent early life stress using a similar MS protocol (Daniels et al., 2004).
In addition, SD-MS rats also showed decreased immobility time in the forced swim test but did not change the preference for saccharin compared to briefly separated rats SD-HD. Accordingly, decreased immobility in the forced swim test could also be considered an increased anxiety-like response or a non-adaptive response to stress, as previously suggested (Anyan & Amir, 2018). Therefore, these results support the hypothesis that ELS induced by prolonged MS during the stress-hyporesponsive period could sensitise or program the neuroendocrine HPA axis and behavioural responses to stress during adulthood in rats (van Bodegom et al., 2017; Wang et al., 2020), affecting anxiety-like behaviour and stress resilience or coping, but it does not seem to clearly increase depression-like behaviour as measured by the forced swim and saccharine preference tests. Probably, differences in sensitivity to MS effects by different rat strains and the different MS protocols and the control group used (unhandled or briefly separated) would be important factors to explain the differences reported across studies.
Although the diet induced major changes in the gut microbiota, we observed specific changes in the microbial composition of rats fed a standard diet with and without MS. The SD -MS group showed higher relative abundances of Lactobacillaceae and lower proportions of Rikenellaceae, Rhodospirillaceae, and Desulfovibrionaceae families, with the former and latter families being the discriminant taxa when both groups of rats fed the standard diet were compared. Interestingly, it has been reported that ELS induced by MS in male rats is associated with higher levels of Lactobacillus like we found, which is associated with decreased social interaction and body weight gain, and increased intestinal permeability (Rincel et al., 2019).
In contrast to the anxiety- and depression-like behaviours found in control-handled rats fed the HFS diet, prolonged MS exposure to ELS seemed to partially counteract the above-mentioned adverse effects of the HFS diet. Rats fed the HFS diet and exposed to early MS had similar outcomes in the elevated zero-maze and forced-swim tests compared with the group fed the SD and briefly separated (and handled) during the same early period. Thus, there was a partially compensatory effect between both factors (MS and HFS diet) because as anxiety levels measured in the EZM (time in open sectors) was similar between SD-MS and HFS-MS groups, and anhedonia scores in saccharin preference test were similar between HFS-MS and HFS-HD groups. Other studies have reported similar results using a HFS diet (40% kcal from fat) in Wistar rat dams from gestation to postnatal day 21, with offspring exposed to ELS by MS without showing anxiety-like behaviour during adulthood (Rincel et al., 2016). The mechanisms involved are not fully understood; however, a possible explanation is that the higher palatability of HFS diets could exert an anxiolytic effect on lactating dams by promoting increased maternal care related to normal HPA axis maturation in stressed rat pups (Rincel et al., 2016). However, other metabolic parameters, such as body weight gain and adiposity in rodents perinatally fed a high-fat diet act synergistically with ELS exposure (Romaní-Pérez et al., 2017) as shown here.
Regarding the effects of ELS on gut microbiota composition, MS also interacted with the HFS diet, restoring some of the alterations in microbiota composition caused by independent application of these factors. Accordingly, we have found that statistically significant differences between SD-HD and HFS-HD groups (such as in Prevotellaceae, Rickenellaceae, Lactobacillaceae, Erysipelotrichaceae, Rhodospirillaceae or Desulfovibrionaceae, among other families) were not found when SD-HD and HFS-MS groups were compared. In addition, ELS by MS has been reported to alter microbiota composition and is used as a model of irritable bowel syndrome in rats (Enqi et al., 2021; O’Mahony et al., 2020).
Remarkably, increased short-chain fatty acid (SCFAs) levels were observed in the MS group compared with the other groups. It is noteworthy that SCFAs possess anti-inflammatory properties and can modulate mood and anxiety; patients with MDD show lower faecal SCFAs than controls, and butyrate seems to have antidepressant properties in mice with anhedonia and cognitive impairment (Silva et al., 2020). Conversely, high faecal SCFAs levels are associated with changes in microbiota diversity, gut permeability, and obesity (de la Cuesta-Zuluaga et al., 2019). In addition, chronic social stress in mice increased faecal acetate and total SCFA levels, which are associated with increased body weight (van de Wouw et al., 2018). Moreover, we previously found that early MS leads to increased neuroinflammation in adult male rats, with increased levels of proinflammatory cytokines in the prefrontal cortex (González-Pardo et al., 2020), suggesting a role for inflammation in abnormal brain maturation and behaviour induced by ELS.
The study of the gut microbiota in the present study showed a large impact of diet, with some changes observed even at the phylum level. Moreover, ELS by MS also had a significant effect on microbiota composition at the phylum level. Although it was more limited than diet, it had a significant effect on some microbiota metabolites. Mirroring the observations of the behavioural data, the microbiota composition was similar between the SD-HD and HFS-MS groups.
The alterations in gut microbiota in both groups fed the HFS diet may be linked to obesity and overweight because the HFS diet combined with ELS has been demonstrated to be related in other studies (Maniam et al., 2016; Tsan et al., 2021). However, the link between obesity and overweight and gut microbiota is bidirectional, varying the directions of this link (Abenavoli et al., 2019) although we were not able to demonstrate it. While the role of microbiota in the beneficial effects of exposure to ELS on behavioural alterations induced by an HFS diet remains to be elucidated, these results suggest that the microbiota-gut-brain axis could be associated with abnormal brain maturation and stress response (Adan et al., 2019).
In conclusion, our results suggest that the combination of these two factors (exposure to the HFS diet and MS) modifies the effect of the HFS diet alone on behaviour and microbiota composition, producing a partial compensatory mechanism that reverses the negative effects induced by each factor independently. The main limitation of our study is that its descriptive nature does not permit the discarding or confirmation of the role of microbiota composition in the behavioural changes associated with diet and ELS exposure because the bond between behaviour and changes in gut microbiota is of correlative nature. It is not clear if behaviour modifies microbiota, or alterations in microbiota modifies behaviour. Moreover, as another limitation, it was performed only in male rats and females should not be excluded. Future studies are needed to establish causality between nutrition, stress exposure, microbiota composition, and mental health and to unravel the complex mechanisms involved (Adan et al., 2019). Additionally, the implication of probiotic administration to restore microbiota composition in future studies could contribute to better understand the links between diet, early adversity and anxiety-like and depression-like behaviour both in rodents and humans, as other studies have shown (Liu et al., 2019).