INTRODUCTION
Mechanical loading increases bone mass by activating osteoblast metabolic activity and proliferation via cellular biomechanical pathways. These pathways are studied in vitro in models of cellular mechanical stimulation [1, 2, 3]. The widely used experimental methods for this purpose utilize stretching of cells adherent to elastic membranes or implementation of controlled external fluid flow to cell cultures [1, 2, 3]. In both, the mechanism of cellular activation is due to shearing forces and subsequential cellular deformation, which causes cytoskeletal activation, mainly involving the microtubular and microfilament components [4, 5]. These experimental methods require sophisticated hardware with essential special handling and complex experimental protocols, but usually unable to produce uniform stretching forces on all the cells in culture, and commonly restricted to the mechanical loading frequencies up to 5Hz [6].
Previously a more versatile experimental approach has been proposed, which is highly reproducible and with much wider range of mechanical frequencies that can be applied on the cultured osteoblasts in monolayer. This method is based on the application of controlled vibration force in a defined range of mechanical parameters [7].
In this model, the cellular deformation is caused by shearing forces, applied by extra- an intracellular fluid flow, which is induced by the one-dimensional accelerated vibration movement of cells adherent to a plastic surface. Since the cells in this model are in the same environmental condition, they exposed to the same magnitude of force during the vibration application on a culture well plate. Therefore, large numbers of cells can be studied in the same mechanical conditions, which are determined by the dimensions of the well plate and its weight, its surface biophysical properties, volume of the culture media in the wells and by a profile of the force generated by a vibration actuator. Additionally, this experimental setup can be used for application of vibration in higher frequencies, i.e., above the infrasonic range (20-70 Hz).
There are several previous reports that human osteoblast in vitro can be stimulated by the high-frequency vibration frequencies [4, 7, 8], probably by mimicking the natural influence of resting muscle on an attached bone, as it is seen on the vibromyogram [9] following muscle's spontaneous periodic contraction in this range of frequencies. These reports are also supported by the in vivo studies that showed an enhancing effect of vibration in this range of frequencies on the bone mass buildup [10].
It has been shown that different sub-ranges of effective vibration parameters for osteoblast proliferation and metabolic activity stimulation exist and not overlapping [7]. The main difficulty that evolved in that model was to keep a constant pattern of vibration parameters and to record these values directly from the moving supporting well plate due to mechanical instability of the electromagnetic actuator and the difficulty of the fine-tuning of the mechanical parameters in this range of frequencies. The source of the variability of vibration signal was the surface friction of the moving parts, i.e., in that model, it was impossible to effectively eliminate or reduce the additional vibration frequencies in the higher or lower range, which were produced by the vibration actuator and by the well plate movement. Because the optimal range of vibration frequencies for osteoblast activation is narrow, the delivery of defined stable external vibration signals is crucial. Additionally, to claim that a specific range of vibration frequencies has an enhancing effect on cultured osteoblast, a close to "pure" sinusoidal vibration force pattern should be applied. Therefore, to determine the role of mechanical excitation of osteoblast by high-frequency vibration, we developed an experimental model that utilizes a low friction system to deliver vibration force to a cell culture well plate. This system provides a relatively stable sinusoidal vibration force that can be effectively controlled and measured.
We hypothesize that by using the designed method of high frequency vibration application, we will be able to provide reliable evidence that human osteoblast is stimulated for enhanced proliferation and will determine the range of on the effective mechanical parameters that trigger this effect.
METHODS
CELL CULTURE PROTOCOL
We studied cultured human osteoblast-like cells primary explant cultures from samples of cancellous bone harvested during knee arthroplasties from distal femurs. The method was approved by the institutional Ethical Committee (No. 1240A). Every donor provided written informed consent. Samples of cancellous bone (2-3 grams each), were incubated in osteogenic media ( DMEM with heat-inactivated fetal calf serum (10%), 2mM L-Glutamine, 100mM Ascorbate-2-Phosphate,20mM HEPES buffer, 10nM Dexamethasone, 150m/ml Streptomycin ,50 U/ml Penicillin) at 37oC within the humidified atmospheric environment of 95% air with 5% CO2 (v:v ) for 20 - 30 days. The cells grow adherent to the plastic tissue culture plates. This type of cells has specific osteoblast-like characteristics, i.e. expression of alkaline phosphatase, synthesis of osteopontin, polygonal multipolar morphology, positive Von Kossa staining, synthesis extracellular matrix with predominantly type I collagen and small amounts of collagen type III and V, non-collagenous proteins like sialoprotein (BSP) and osteocalcin. Additionally, these cells demonstrate matrix mineralization in vitro and bone formation in vivo [11, 12, 13]. The cells were passage into 24 well-plates. Each well is seeded with 2×103 cells.
MECHANICAL SYSTEM CONFIGURATION
Plastic 24 well-plate with the cultured osteoblast-like cells (weight 120 grams including the culture media), was mounted on a stage that moves on low friction support (Figure 1). The horizontal movement of the stage with the attached well plate is controlled by an electromagnetic actuator. A piezoelectric accelerometer (accelerometer sensitivity = 100mV/g) is attached to the moving stage with a solid connection to well-plate in the horizontal direction of movement. The relation between the output voltage from a piezo electric accelerometer and the acceleration is linear throughout frequencies of vibration of 10-80 Hz and at the displacement amplitude of up to 30mm. This is an open loop, externally controlled setup.
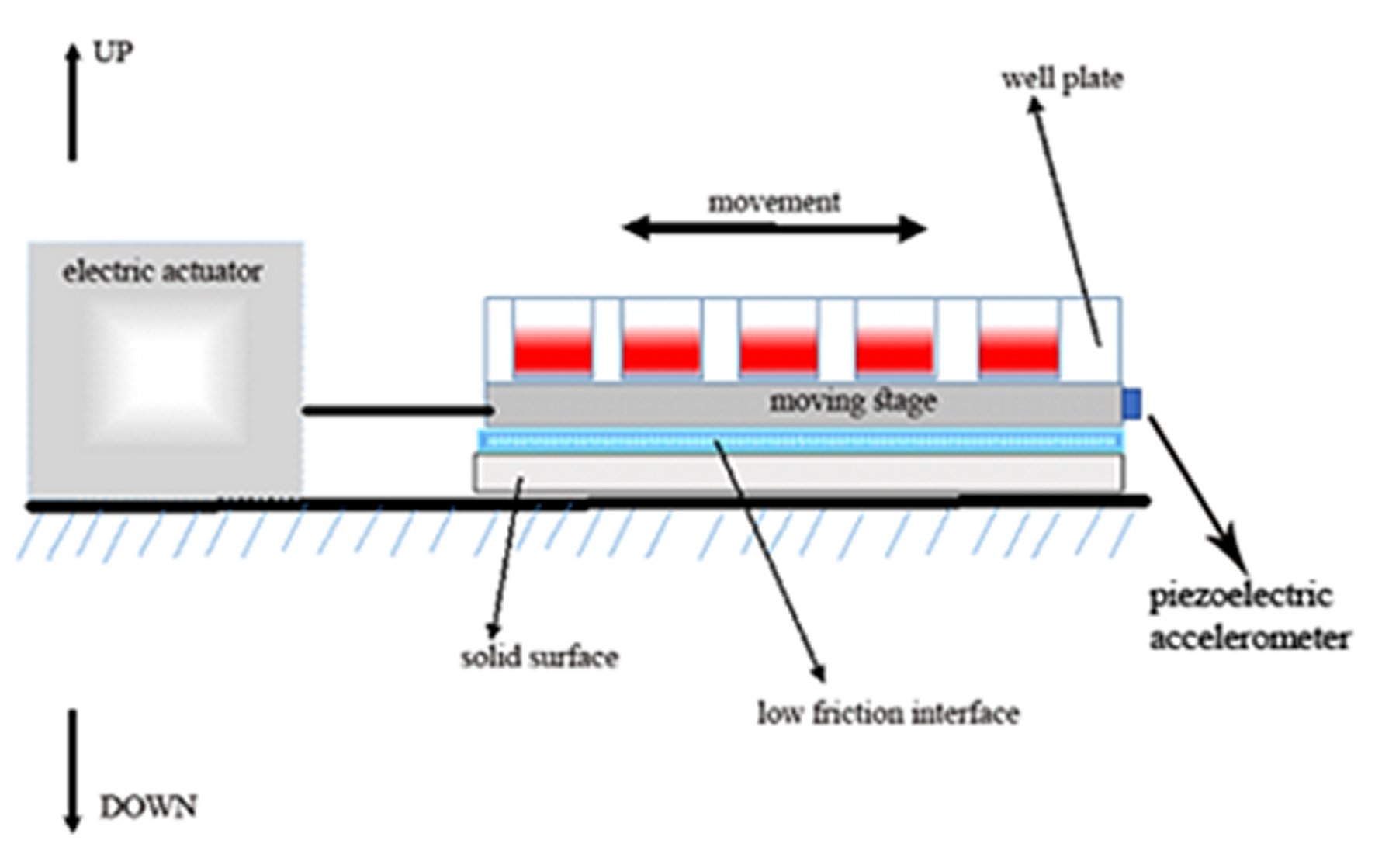
Figure 1. The experimental arrangement (schematic representation). A 24 well-plate containing cells adherent to the plastic surface, covered with culture media, and is tightly attached to the moving stage on a low friction interface. The stage movement is controlled by an electric actuator. The acceleration and displacement of the stage during vibrating movement are recorded by an accelerometer, attached to the stage in a horizontal direction.
This system produces a vibration movement, which is near sinusoidal, with maximal displacement amplitudes of 25±5 mm and a near sinusoidal vibration frequency of 10 and 60 Hz (Figure 2). We defined the dominant and additional harmonic frequencies of the moving well plate from the recordings of the piezoelectric accelerometer (Figure 2). Through a double integration, the displacement of the well plate movement is measured. According to the detected spectra of the vibration applied by this method it was revealed that when the actuator is tuned to 20 Hz of vibration, 70% of the frequencies are within the range of 10 - 30 Hz (peak to peak acceleration ≈ 0.02g), and when the system is tuned to excitation of 60 Hz vibration, 98% of the frequencies are within the range of 50 - 70 Hz (peak to peak acceleration ≈ 0.03g).
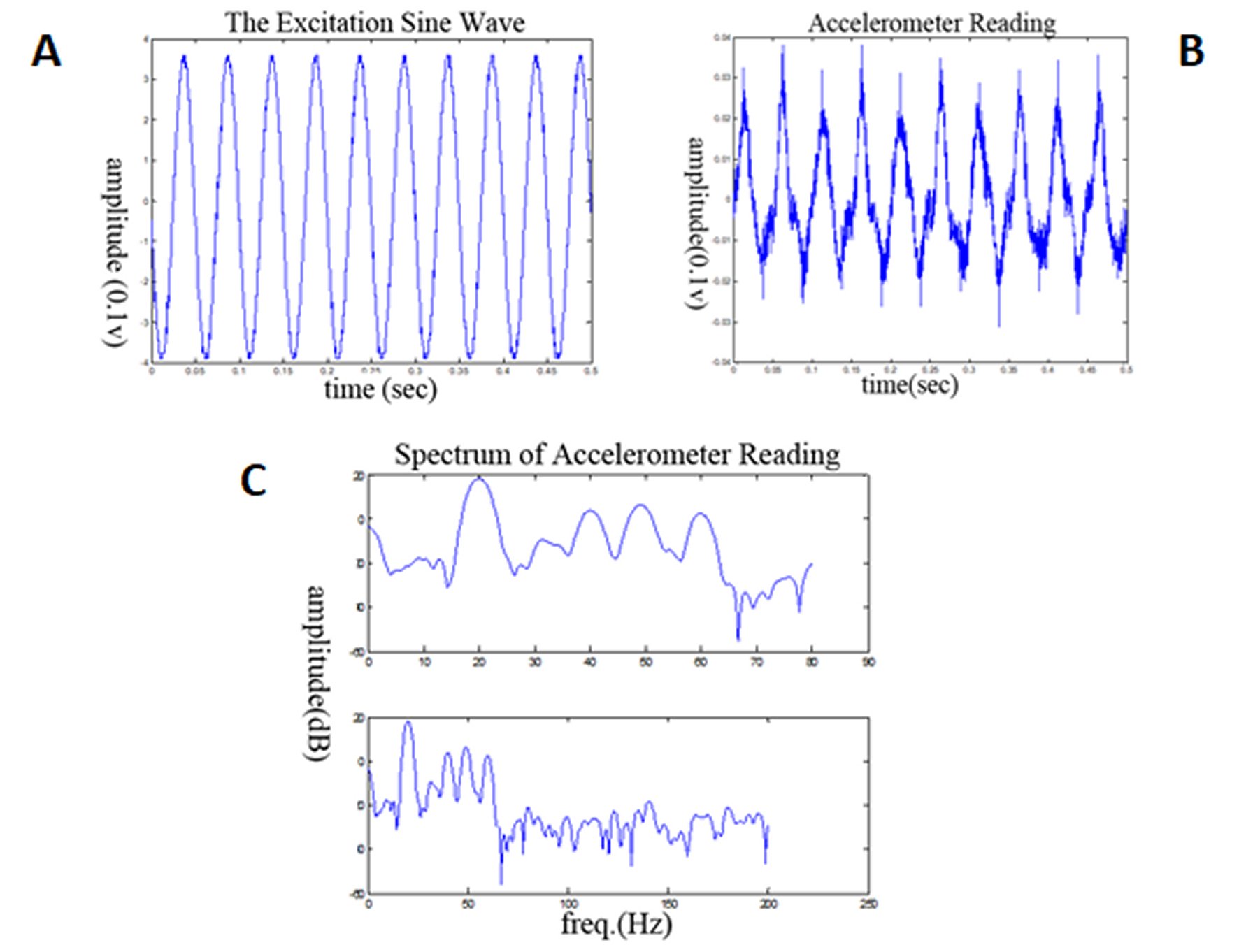
Figure 2. Illustrations of the 20 Hz excitation profile delivered by the actuator (A) and of the accelerometer recording from the moving stage (B).The accelerometer readings reflect the actual mechanical force acting on cells, which are adherent to the moving surface, and appears to be close to similar to the excitation signal from the actuator (amplitude readings represented in V×10-1). The maximal amplitude of displacement is 25±5mm. The spectra of the vibration frequencies, which were applied to the cells (C) shows that 70% of the frequencies, up to 70 Hz frequency, is in the range of 10-30 Hz.
MECHANICAL STIMULATION PROTOCOL OF THE CULTURED OSTEOBLAST-LIKE CELLS
Following first passage of cells, replicates of an equal number of cultured cells were submitted to the excitation conditions of 20Hz or 60 Hz. The applied protocol of cultured replicates to mechanical excitation was corresponding to the previous similar studies [4, 7] and utilized two-minute exposure repeated four times at 24 hours intervals. The doubling time of osteoblasts relies on the seeding density. When seeded at the range of 103 cell/cm2 density (as during this study), the doubling time is around 10 hours [14]. Therefore, four days of the experiment (96 hours) represent 10 doubling periods. At this time, the cell culture is near 2D confluence, and also the proliferation rate ceases, and the cells start with higher metabolic activity in matrix elaboration. Therefore, within the period of 4 days, the cells reach the maximal proliferation rate. Accordingly, we evaluated the results on this point in time of the cell culture growth.
The culture samples kept in static conditions, without exposure to vibration protocols, we used as controls. Eight cell culture samples for every experimental condition were studied.
The number of cells in each culture sample was estimated by direct counting in low-power microscopic field. The average of cell numbers from three different microscopic fields in each culture sample, after exposure to mechanical stimulation and in control samples, was recorded. This method of cell number measurement doesn't require interference with the culture conditions.
CELL DEATH AND PROLIFERATION
LDH activity within the culture media, a marker of cell death because of LDH leakage via damaged cell membranes, was measured in the samples exposed to mechanical vibration and in the static controls. Briefly, LDH activity in culture media samples was determined by 340 nm wavelength spectrophotometry of the reduced nicotinamide adenine dinucleotide (NAD), which is directly proportional to LDH activity [15, 16].
Accordingly, the cell proliferation comparison among the studied samples in different conditions, i.e., exposed to 60 Hz, 20 Hz of vibration protocols and static controls, was based on as a result of the deduction of cell death change from the total cell number in the culture samples.
CELLULAR MATURATION
Cellular alkaline phosphatase activity, a marker of osteoblast maturation from progenitors, increases in the Stage 2 osteoblast cell cycle after the Stage 1 cell proliferation ceases [17].
The cellular alkaline phosphatase activity was measured by 410 nm wavelength spectrophotometry [18] after microscopic cell counting, utilizing cell lysate following incubation with P-nitrophenyl phosphate substrate.
DATA ANALYSIS
The comparison of two parameters was made by the t-test and the comparison of three parameters by ANOVA, and subsequentially by the Dunnett's post hoc test (following verification of normal distribution of results and with a p-value below 0.05). A P-value of less than 0.05 was considered as a significant difference between the compared values. The calculations of the statistical comparisons were done using SigmaStat software (version 2, SPSS Inc., Chicago, Il, USA).
RESULTS
At the 60 Hz vibration the number of cells significantly increased in comparison to control static condition (average 68±4 SEM cells/microscopic field vs. average 55±4 SEMcells/microscopic field respectively, p<0.05, one-way ANOVA, n=8, Figures 3 and 4). No significant change in number of cells exposed to 20Hz protocol in comparison to static controls was observed (average 64±3 SEM cells/microscopic field vs. average 55±4 SEMcells/microscopic field respectively, p>0.05, one way ANOVA, n(controls)=8, n (20 Hz)=12, Figures 3 and 4).
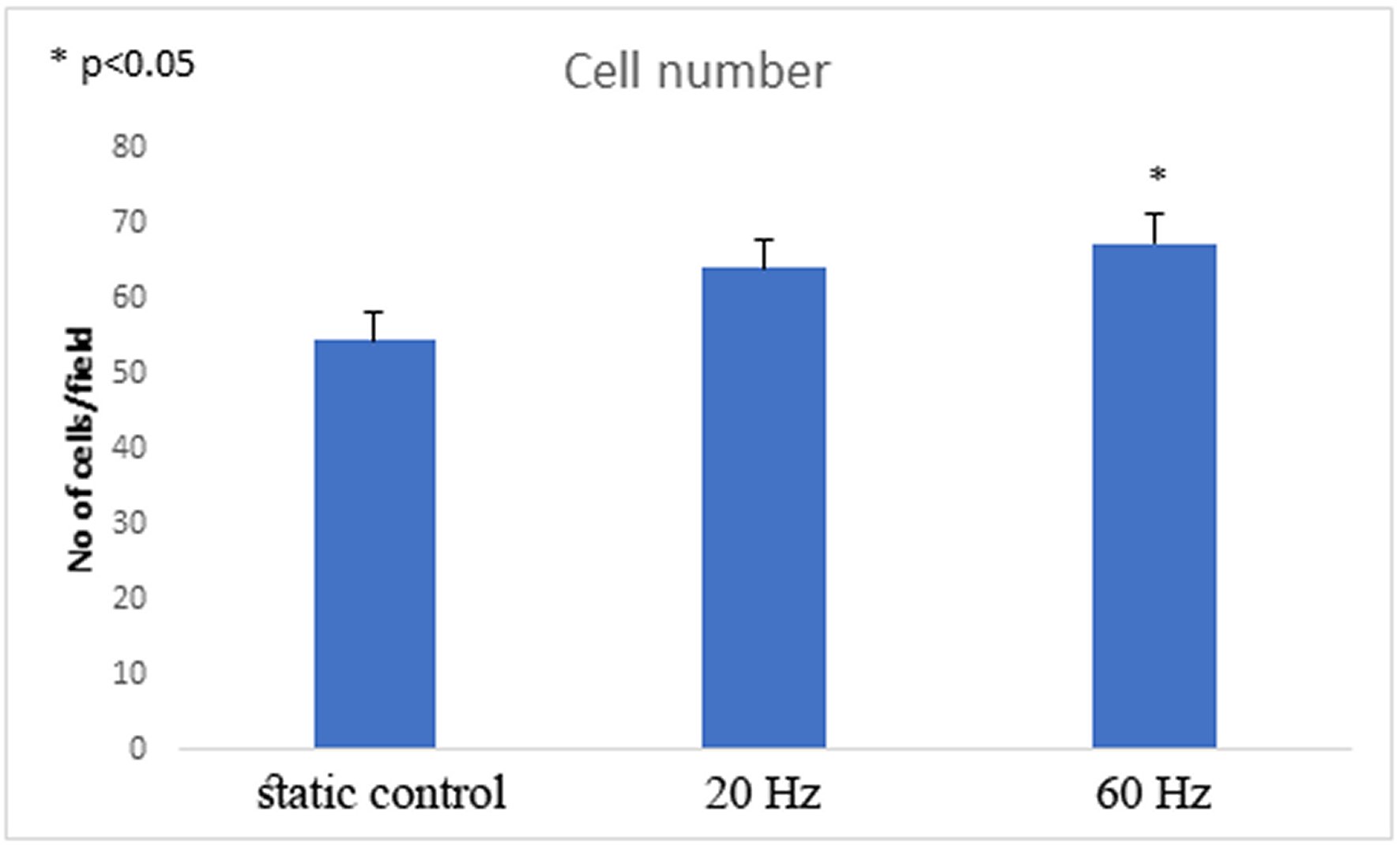
Figure 3. The number of cells rises significantly following 60 Hz excitation (average 68±4 SEM cells/microscopic field vs. average 55±4 SEM cells/microscopic field in controls, p<0.05, n=12).
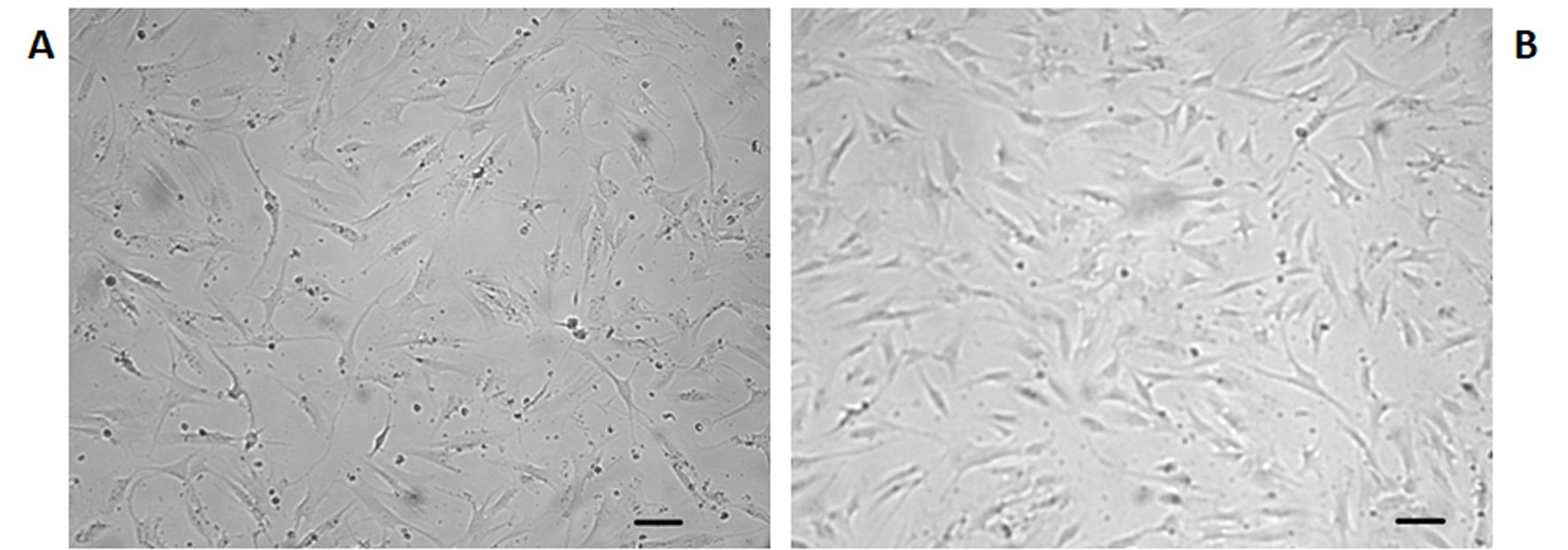
Figure 4. Representative micrographs of osteoblasts in culture on the fourth day of the experiment (Scale 20 µm).A: Cells kept in static conditions. B: Cells after exposure to 60 Hz vibration protocol. More cells in B are apparent.
There was no difference in the media LDH activity in cultures exposed to either 20Hz or 60Hz vibration protocols and the static controls (average 163 ± 9 SEM U/L and average 167±8 SEM U/L vs. average 193±12 SEMU/L respectively, p>0.05, one-way ANOVA, n=12).
A significant decrease in cellular alkaline phosphatase activity following 60 Hz vibration protocol was found (average 0.559±0.041 SEMU/L/cell in the microscopic field vs. average 0.794±0.0571 SEMU/L/cell in microscopic field in static controls, p<0.01, t-test, n=12, Figure 5).
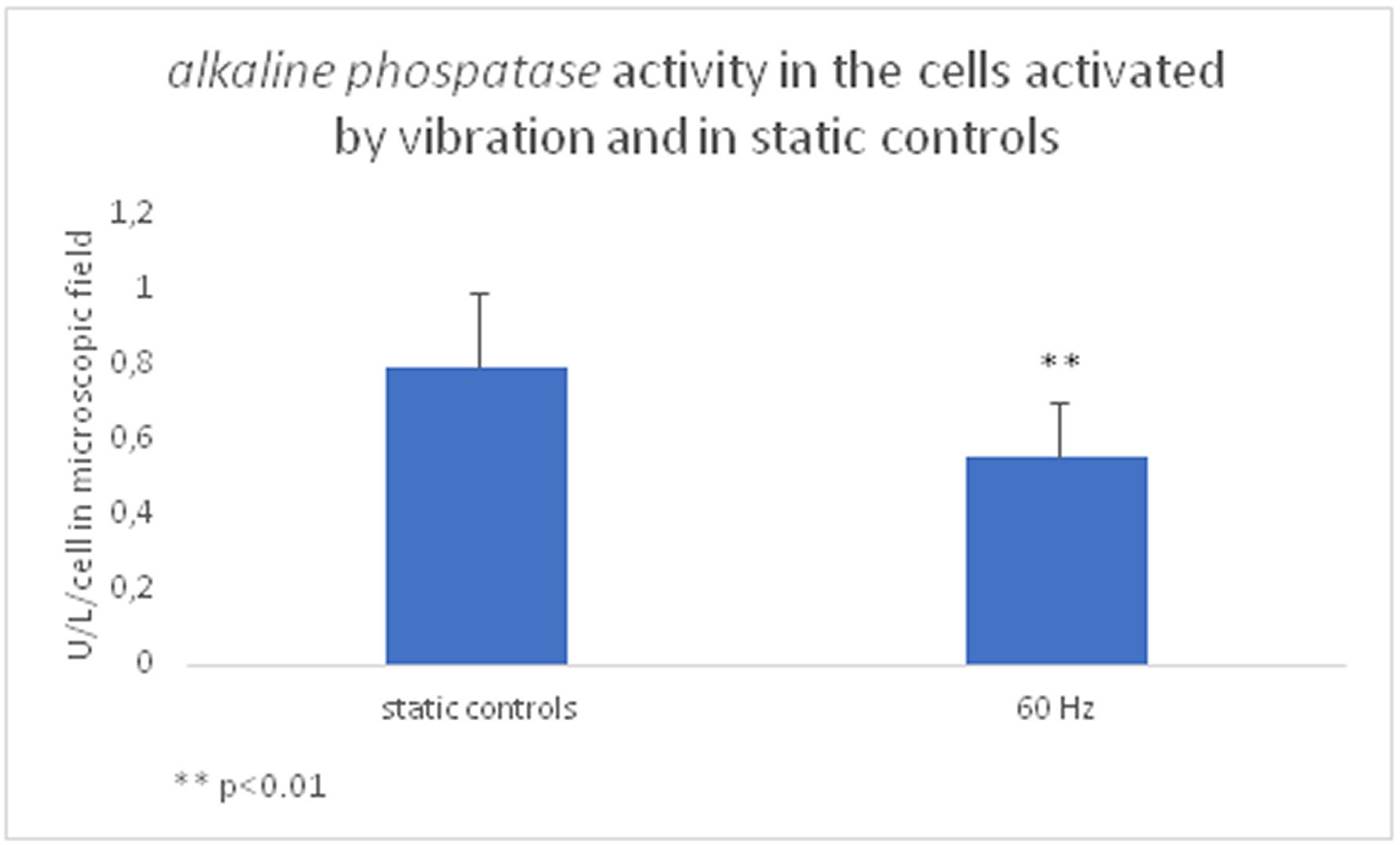
Figure 5. After the 60 Hz excitation, the cells' maturation state decreased -significantly lower alkaline phosphatase activity in the mechanically activated cells was found (average 0.559±0.041 SEM U/L/cell in the microscopic field vs. average 0.794±0. 0571 SEM U/L/ cell in microscopic field, p<0.01, n=12).
DISCUSSION
In this study, we determined the narrow range of high frequencies spectrum of 50-70 Hz mechanical vibration (when excitation by 60Hz provided by the actuator) that is effective in enhancing human osteoblast proliferation in vitro. This conclusion is following the deduction of cell death rate from cell numbers per sample in different mechanical protocols that were studied, i.e., 60 Hz excitation of cells caused a significant increase in cell number in comparison to controls, without a change in cell death (expressed by LDH activity in culture media) meaning that an enhancement in cell proliferation has occurred. This phenomenon was not apparent following 20Hz excitation. Furthermore, this conclusion is independently supported by the evidence of the lower maturation state of the cells exposed to the 50-70 Hz mechanical vibration protocol as expressed by lower alkaline phosphatase cellular activity. We preferred this double approach of independent assays to increase the confidence in the results. The results show that after the exposure to the around 60 Hz excitation, the cells react by increased proliferation and stay in a lower differentiation state, as expected. This effect doesn't happen in the lower range of the vibration parameters (cellular excitation by 20 Hz that provide 10-30 Hz of vibration spectrum), i.e., by this excitation protocol neither cell number no LDH activity in culture media were not significantly different from the cells kept in control static environment. This fact supports our basic hypothesis that above 10 Hz of mechanical cellular excitation only a specific band of frequencies of vibration elicits a proliferation response.
This observation is different from the previous report on a more pronounced proliferation response by 20Hz excitation of human osteoblasts [7], but similar to a previous report on enhanced osteoblast proliferation following 45 Hz vibration protocol [19]. We attribute this controversy to the differences in the experimental setups in these previous reports in terms of acceleration magnitude and direction, time of the vibration protocols applications, and the methods of mechanical measurements. In the present study we determined the narrow range of mechanical frequency of high-frequency horizontal vibration, which causes the highest rate of cell proliferation in human osteoblast that has not been defined precisely till now.
The mechanotransduction in osteoblast involves numerous cellular pathways that propagate mechanical force from the extracellular milieu and translated to cellular synthetic response. The exact interrelation of these pathways is not sufficiently clear and is under extensive research efforts. Overall, this process is initiated by the external mechanical force causing shear stress on the cellular membrane and governed by the mechanical stiffness properties of the supporting extracellular matrix. The deformation of the cellular membrane affects the cell membrane potential by inducing electrical currents of extremely low electromagnetic intensities (0.02-0.03 mT) via cellular membrane by a flux of calcium and phosphate ions [20, 21, 22]. Additionally, the external force is transferred via transmembrane molecular extensions (Integrins, Cadherins, etc.) and cytoskeletal components to a nuclear Lamin A with a subsequential transcription activity [23].
In this study, we did not attempt to determine the specific mechanotransduction biomechanical pathways that are evoked by this mechanical excitation method, but rather provide the basic optimal biomechanical data for the further mechanotransduction research by the presented experimental setup that generates a uniform force transfer to a large number of cells in two-dimensional culture.
The main limitation of this study is the lack of a determination of the possible change of the cell cycle profile following the discovered enhancing proliferative effect of 50-70 Hz mechanical vibration. This important issue can substantially support the presented here experimental results and can be further investigated by the presented experimental setup, which is easily reproducible.
CONCLUSIONS
According to the study hypothesis, we describe a tunable and easily reproducible mechanical platform that can be utilized for the research of mechanotransduction in osteoblasts, with the determination of the optimal mechanical frequencies for induction of cell proliferation. Naturally, other cellular functions related to mechanical stimulation can be studied by using this versatile method.