1. INTRODUCTION
Diabetic retinopathy (DR) is one of the most important complications that develop in patients with diabetes mellitus (DM) and results in vision loss. According to a report by the World Health Organization, there are currently 360 million diabetic patients worldwide. If it continues with the current prevalence rate, it is predicted that there will be about 1 billion diabetic patients in 2030 [1].
DR is one of the leading causes of preventable blindness and is a serious public health problem. With the increase in the number of diabetic patients, the number of patients with the diagnosis of DR, which is one of the complications related to this disease, is also increasing [1]. DR is diagnosed by the clinical manifestations of vascular abnormalities in the retina. Clinically, DR is divided into two stages: non-proliferative DR and proliferative DR [1]. Non-proliferative DR represents the early stage of DR, where increased vascular permeability and capillary occlusion are the two main observations in the retinal vasculature. At this stage, retinal pathologies such as microaneurysms, hemorrhages, and hard exudates can be detected by fundus photography, although patients may be asymptomatic. Proliferative DR, a more advanced stage of DR, is characterized by neovascularization. At this stage, patients may experience severe visual impairment when new abnormal vessels leak into the vitreous (vitreous hemorrhage) or when tractional retinal detachment is present [2].
High mobility group box proteins are non-histone nuclear proteins with multiple functions in the cell. A 30 kDa long protein binds chromatin in eukaryotic cells and is secreted from eukaryotic cells [3]. It is 215 AA long and consists of 3 parts consisting of 2 DNA binding regions (Box-1 and Box-2) and a C-terminal region [4]. HMGB-1, HMGB-2, HMGB-3 is a member of the HMGB protein family. While expression of HMGB-2 and HMGB-3 is limited to a certain period of life and some cells, HMGB-1 expression is widespread and persists into adulthood [5]. HMGB-1 acts as a chromatin-binding factor. It binds non-specifically to the minor groove of DNA, binds to some receptors, including p53 and steroid hormone receptors, and modifies the interaction of transcription factors with DNA. It plays a role in DNA repair, transcription, differentiation, extracellular signaling, and somatic recombination [5]. In addition to these nuclear functions, it also functions as an extracellular signal molecule by being secreted passively from necrotic cells and actively from cells involved in inflammation [6]. Important receptors to which it binds extracellularly are; RAGE (receptor for the advanced glycation product), TLR-2 (Toll-like receptors), TLR-4, and TLR-9. RAGE is the receptor for extracellular HMGB-1 that mediates its intracellular effects [7]. Diagnosis of the disease can be made easily in the presence of classical symptoms and complications specific to DM. However, real and early diagnosis is based on the correct use of some laboratory methods and the evaluation of the results [8]. As the incidence of DM increases with the ever-increasing rate of obesity, there is a real need to better understand DM-related retinal damage. In the last decade, the role of retinal inflammation in retinal damage caused by DM has become increasingly important [9]. In addition to specific cytokine effects on the retina, sterile inflammation has recently been observed to cause retinal damage [10]. Sterile inflammation is a pathogen-free form of inflammation that can be caused by trauma, ischemia, or other stressors. High glucose levels associated with DM can activate a type of sterile inflammation [11]. HMGB-1 is the main mediator of sterile inflammation in the retina [12]. Studies in diabetic patients with proliferative retinal disease have shown a significant association between disease severity and increased HMGB-1 levels [13].
Based on these clinical findings, researchers investigated the role of HMGB-1 in the diabetic retina in rodent models. HMGB-1 has been shown to mediate DM-induced damage in retinal pericytes [14] and Müller cells [15]. In another study, it was reported that HMGB-1 plays an important role in inflammation in retinal ganglion cells [16] and retinal pigment epithelial cells [17]. Using diabetic mice, the researchers found that DM increased HMGB-1 levels. They also showed that it decreased when glycyrrhizin, an HMGB-1 inhibitor, was used [18]. In another study, silencing HMGB-1 in cells was shown to decrease apoptosis and inflammatory mediators [19].
We believe that HMGB-1 protein is a promising prognostic marker for predicting the course of retinopathy and the prognosis in patients with DR. The aim of this study is to investigate whether serum HMGB-1 protein levels can be used as a biomarker about the severity of the disease in patients with diabetic retinopathy.
2. MATERIAL AND METHODS
This study was carried out in the internal medicine and ophthalmology departments of the Gaziantep Medicalpark Hospital. The records of 50 patients diagnosed with type-2 DM with DR and 47 healthy subjects who applied to our clinic between January 2016 and December 2018 were retrospectively scanned. All procedures followed the Declaration of Helsinki rules, and written informed consent was obtained from all patients. Local ethics committee approval was obtained (Ethics Committee for Clinical Trials of SANKO University, 07/2018). Patients who were followed up with the diagnosis of type-2 DM and who were diagnosed with DR during routine eye examinations were included in the study. A complete ophthalmic examination, including best-corrected visual acuity (BCVA), slit-lamp biomicroscopy, and fundoscopy, was performed for all patients. The diagnosis of DR was made according to the biomicroscopic fundus examination. Patients with thyroid-related diseases, rheumatologic diseases, a history of any eye surgery, ocular trauma, ocular inflammations containing uveitis, and type 1 DM were excluded from the study. Patient files were scanned, and information such as age, gender, and results of routine laboratory tests was obtained retrospectively.
Five cc of venous blood was taken from the patients, and control groups using a biochemistry tube containing clot activator and separator for routine laboratory tests and serum HMGB-1 levels to be studied under appropriate conditions. Blood samples were centrifuged for 8-10 minutes at 4000 rpm within 1 hour of collection. The samples were taken at -80 °C and kept at 4 °C in a refrigerator one night before the measurements. After defrosting, the samples were mixed using a vortex and added to microplate wells without losing time. The measurements were performed using the ELISA method, repeating it twice for each sample. Human HMGB1 levels were measured quantitatively by the manufacturer instructions of the Bio-Techne brand commercial kits (Bio-Techne Ltd., Abingdon-Oxfordshire, United Kingdom) with catalog number NBP2-62766. The analysis was performed using the double-antibody sandwich enzyme immunoassay technique. All concentration/absorption graphic curves of the human ELISA tests and calculations regarding the results were performed on the program of the Biotek_ELx808 (Winooski, Vermont, USA) device. The test for human HMGB1 had a sensitivity of 8.57 pg/mL and a detection range of 2-2000 pg/mL. Intra-assay and inter-assay coefficients of variation were 8.2% and 10.4% respectively [20].
The study was performed in accordance with the Declaration of Helsinki. Informed consent was obtained from all patients and the local ethics committee (Ethics Committee for Clinical Trials of SANKO University, 07/2018) gave ethical approval.
2.1. STATISTICAL ANALYSIS
Statistical analysis was performed with Statistical Package for Social Sciences (SPSS) 21.0 for Windows (SPSS Inc., Chicago, IL, USA). Frequency, percentage, and mean ± standard deviation (SD) were used as descriptive statistics. G-Power software is used for power analysis and sample size calculation. The Kolmogorov-Smirnov test was used to determine whether the distribution of the data followed a normal distribution. P>0.05 was considered a normal distribution in the Kolmogorov-Smirnov test. Student’s t-test was used to compare the means of two normally distributed independent samples, and the Mann-Whitney U test was used when there was no normal distribution. Spearman rank correlation was used to measure the degree of association between two variables. P<0.05 was considered statistically significant.
3. RESULTS
A total of 97 subjects were included in the study. Of the 97 subjects, 50 had type 2 diabetes-related DR, and 47 were apparently healthy. Demographic characteristics and blood test parameters of the patients are presented in Table 1. Of 50 diabetic patients, 18 were men (36%), and 32 were women (64%). Of 47 healthy control subjects, 22 were male (46.8%) and 25 were female (53.2%). The mean ages were 62.38±8.69 and 59.34±7.22 years for the diabetic and control groups, respectively. There was not a significant difference among the groups in terms of sex (p=0.2) or age (p=0.06). Mean serum HMGB-1 levels were 24.99 (4-181) and 12.60 (3-27) pg/mL in the DR group and control group, respectively. There was a significant difference among the groups in terms of HMGB-1 levels (p<0.001). A comparison of serum HMGB-1 levels between patients and the control group is presented in Table-2. In the Spearman correlation analysis between blood glucose and HMGB-1 levels in the DR group, there was a positive correlation between blood glucose and HMGB-1 (Spearman's rho (ρ)=0.07). Similarly, there was a positive correlation between HbA1c and HMGB-1 levels (Spearman's rho (ρ)=0.06). As a result, it was determined that HMGB-1 levels increased as blood glucose and HbA1c levels increased. The correlation between HMGB-1 values and clinical data in DR patients is presented in Table 3. In the ROC analysis of serum HMGB-1 levels in the DR and control groups, a cut-off value was determined. Accordingly, the cut-off value was found to be 18.25 pg / mL (Figure 1).
Table 1. Demographic and clinical data of patients.
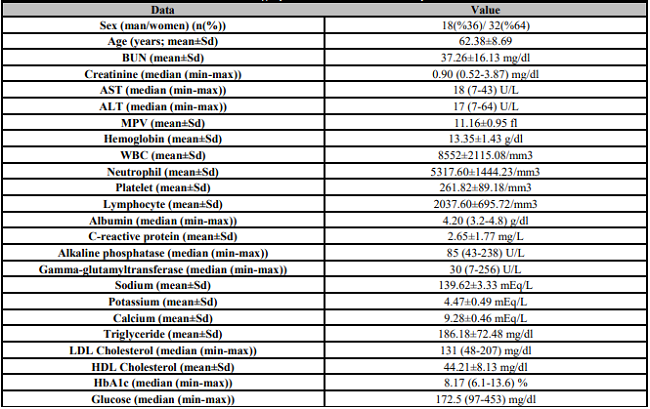
BUN: Blood urea nitrogen; AST: Aspartate transferase; ALT: Alanine transaminase; MPV: Mean platelet volume; WBC: White blood cells; LDL: Low-density proteins; HDL: High-density proteins.
Table 2. Comparison of serum HMGB-1 levels between patient and control groups.

*The Mann-Whitney U test,
**Student t test.
4. DISCUSSION
DR is one of the most common microvascular complications of DM. Progressive inflammation can lead to severe vision loss through the formation of neovascular changes [21]. The mechanism of development and progression of DR is quite complex and has not yet been fully understood. In the literature, there are many publications examining and explaining the roles of vascular endothelial growth factor (VEGF), insulin-like growth factor (IGF)-1, and tumor necrosis factor (TNF) in the DR process [22]. In recent years, the functions of a new protein, HMGB-1, have gotten a lot of attention in the DR process. HMGB-1 is a nuclear protein. Extracellular release occurs either actively by cytokine stimulation or passively by necrotic cell death [23]. It has been shown in various studies that HMGB-1 levels are significantly increased in sepsis, rheumatoid arthritis, and various types of cancer [7, 24]. It has been reported that there is a significant relationship between increased HMGB-1 levels and mortality in sepsis patients and a significant relationship between HMGB-1 levels and metastatic spread and invasion in patients with various types of cancer [25]. Elevated levels of HMGB-1 have been reported in diabetic patients in the literature. Devaraj et al. stated that they detected high levels of HMGB-1 in the blood circulation of patients with type 1 DM [26]. According to the study of Hagiwara et al., serum HMGB-1 levels were increased in the experimental rat model in which hyperglycemia was induced by glucose infusion [27]. In our study, we compared serum HMGB-1 levels in patients with DR, known to develop based on DM-related inflammation, with those in the control group. We showed that HMGB-1 levels were significantly increased in the patient group with DR, consistent with the literature. In addition, we showed that as blood glucose and HbA1c levels increased, HMGB-1 levels increased in parallel. Fu et al. found that serum HMGB-1 levels in diabetic patients increased in high glucose conditions, which was consistent with our findings [28]. Similarly, Dasu et al. reported that type-2 diabetic patients have high levels of HMGB-1 in their blood circulation [24]. El-Asrar et al. compared HMGB-1 levels in vitreous samples from patients with proliferative DR and patients without DM and found it significantly higher in the group with proliferative DRP [29]. In a different study by the same authors, it was reported that higher HMGB-1 levels of vitreous samples in the group with proliferative DR compared to the non-diabetic group. In addition, the sub-group with severe hemorrhages and neovascular findings in the proliferative DR group had higher vitreous HMGB-1 levels compared to the sub-group with proliferative DR of low severity [13]. According to this study, the increase in HMGB-l levels was interpreted as giving clinicians an idea about the severity of DR. Shen et al. compared serum and vitreous HMGB-1 levels in patients with proliferative DR and in the control group and reported that both were found to be significantly higher in patients with proliferative DR [30]. Wang et al. compared the plasma HMGB-1 levels of patients with type-2 DM and healthy subjects and reported that plasma HMGB-1 levels were higher in the type-2 DM group than in the non-diabetic group [31]. However, since eye examinations of the patients were not performed in that study, the presence or severity of retinopathy, if present, is not known in the diabetic group.
After the relationship of HMGB-1 and DM was demonstrated in many studies mentioned above, research was also conducted on the prevention of diabetic complications including DR by studying the action mechanisms of HMGB-1. HMGB-1 has been suggested as a late mediator of inflammation based on DM [32]. Zhao et al. suggested that HMGH-1 might be a therapeutic target, which may inhibit inflammation and delay diabetic complications including DR [1]. Jiang et al. showed that HMGB1-TLR9 signaling pathway might be a step in the pathogenesis of DR. They reported that blockage of HMGB-1 may be a novel therapeutic approach to treat diabetic retinopathy and other microvascular complications related to DM [33]. Yu et al. reported that HMGB-1 is associated with retinal cell apoptosis in DR [34]. Similarly, Muhammad et al. showed that HMGB-1 causes retinal cell apoptosis via promoting oxidative stress in retina [35]. Fu et al. emphasized that HMGB-1 is as important as vascular endothelial growth factor (VEGF) on the pathogenesis of DR [28].
This study has some limitations. First, HMGB-1 levels were measured in the serum. Measuring the vitreous levels of this protein would make the results more reliable. The limited indication for vitrectomy surgery in patients with non-proliferative DR makes it difficult to obtain a vitreous sample. The major limitation of the study is retrospective cross-sectional study design. In addition, there is no follow-up period including measurement of the levels of these proteins at the time of diagnosis before diabetic retinal involvement and comparing the results among the same patients after diabetic retinal involvement occurs. A comparison of these patients among themselves and with the control group would give the most accurate and rational results.
In conclusion, although the cellular action mechanisms of HMGB-1 have not been clearly understood, evidence that HMGB-1 increases the inflammatory response, promotes angiogenesis, and ultimately causes destruction in DR with different mechanisms is getting stronger with new studies added to the literature, including our study [13]. Our study suggests that HMGB-1 may be a useful biomarker in managing DR, aiding in the prediction and treatment of microvascular complications.