INTRODUCTION
Osteoporosis is defined as a systemic skeletal disease characterized by low bone mass and microarchitectural deterioration of bone tissue with a consequent increase in bone fragility and susceptibility to fracture. Worldwide data indicate that osteoporosis is increasingly becoming a global epidemic: the number of individuals aged 50 years or more at high risk of osteoporotic fracture in 2010 was estimated at 158 million and is set to double by 2040 (1); also, 1 in 3 women over the age of 50 years and 1 in 5 men will experience osteoporotic fractures in their lifetime. Causes of osteoporosis include estrogen or nutritional deficiencies, hereditary or chronic diseases, aging, and long-term exposure to drugs (2).
Current pharmaceutical treatments may cause side effects including several gastrointestinal reactions. Also, drugs that inhibit bone resorption can suppress bone formation too, contributing to the pathogenesis of osteonecrosis (3). That is why there is growing concern in developing nutritional strategies with fewer side effects and greater intake adherence to prevent and assist osteoporosis treatment. These strategies have to include effective and long-term safe food bioactive ingredients with the ability to stimulate bone formation, establishing synergy between food functionality and most suitable nutrients for bone tissue main components.
Bone is a connective tissue composed of an organic matrix (30 % w approx.) made up mainly of type-I collagen (90 % w approx.) and inorganic components (45 % w calcium salts and 25 % w water approx.). Unlike other connective tissues, bone matrix possesses the ability to become calcified in a special way: calcium phosphate biocrystals of hydroxyapatite (Ca10(PO4)6(OH)2) are fixed to collagen fibers (Fig. 1) whose good condition is essential to support bone mineral components (4).
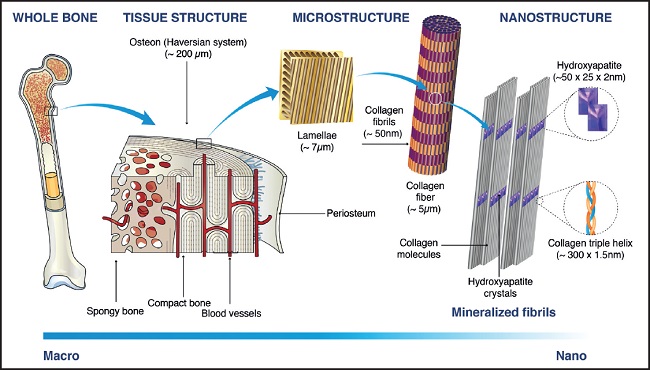
Figure 1. Bone structure hierarchical multiscale levels. Image adapted from Sadat-Shojai M, et al., 2013 (5), with permission
Aging and estrogen secretion decrease at menopause cause a slowdown in collagen synthesis (6). As the bone collagen network progressively deteriorates, calcium salts are released (decalcification) and bones lose mass, becoming brittle and more prone to fracture. Therefore, functional supplementation to improve bone health should take into account providing ingredients to nourish both the mineral and organic parts of the bone.
Traditionally, supplementation based on calcium salts and vitamin D has been recommended to prevent osteoporosis and fractures (7). However, there is much controversy regarding its proper mode of use (8) and it only targets the mineral part of the bone. As a source of calcium, oral hydroxyapatite from animal bone tissues (bovine or porcine) has acquired special relevance since the fact that it is the specific form of calcium phosphate present in bone gives it certain advantages. In fact, it provides a physiological Ca/P ratio, favoring the necessary balance between both minerals to avoid decalcification (9). Furthermore, the affinity existing between bone hydroxyapatite and bone matrix proteins (as evidenced by their bond to build bone nanostructure [Fig. 1]) makes it a compound naturally suitable for formulating protein-mineral complexes, which are not a mere heterogeneous physical mixture, since protein component acts as a binder for mineral components, forming a homogeneous compound (10). Protein-hydroxyapatite complexes provide nutrients for both organic and mineral parts of bone and also, thanks to the binding capacity of proteins, calcium absorption is higher than that of organic or inorganic calcium salts (11).
From the 1970s to the present day, an ossein-hydroxyapatite complex from bovine bone, containing collagenous and non-collagenous proteins and hydroxyapatite, has been commercialized and studied with positive results (12). Following the same line, the product object of this study is also a bone protein-mineral complex but, in addition to micronized hydroxyapatite, its protein component is exclusively hydrolyzed collagen or collagen peptides (neither the entire molecule nor non-collagenous proteins), which have shown high bioavailability (13), calcium chelating capacity (14), and multiple bioactivities (15).
As is known, collagen is the main component of the extracellular matrix in animal connective tissues and the most abundant protein in the human body. Edible hydrolyzed collagen or collagen peptides come from different animal sources (bovine, porcine, fish…) and are obtained by hydrolysis of gelatin or denatured collagen by enzymatic or chemical methods until reaching a molecular weight of less than 6000 Dalton.
Hydrolysis has been shown to improve the functional and nutritional properties of proteins, and collagen peptides have exhibited several physiological activities (15). Among these, it is worth noting its osteogenic capacity, whose mechanism revealed in in vitro studies (16,17) supports the results of in vivo studies (18,19). Furthermore, bone beneficial effects of calcium and vitamin D supplementation increase remarkably if it is accompained by oral hydrolyzed collagen intake (20-22). On the other hand, when calcium intake is clearly deficient, taking hydrolyzed collagen does not produce appreciable bone beneficial effects (23,24). This indicates that to effectively stimulate bone formation through diet supplementation, it is important to cover both the functional and nutritional aspects of bone. The first requires bioactive components that act as biological signals to promote osteoblast activity, and the second protein and mineral components adapted to the metabolic needs of bone. What is more, bringing these components together in the same food supplement presumably allows to take advantage of the synergy between them.
Thus, the present study assesses, for the first time, the in vitro osteogenic activity of a hydrolyzed collagen-hydroxyapatite complex, which combines in a homogeneus food supplement the functionality of collagen peptides with nutrients naturally related to main bone components. In addition, the evaluation is carried out after simulating gastrointestinal digestion and absorption processes using an in vitro methodology for a better approach to in vivo systems.
MATERIALS AND METHODS
SAMPLE
A hydrolyzed collagen-hydroxyapatite food complex (Phoscollagen®, PHC®) manufactured and provided by Protein, S.A. (Girona, Spain) was used for this study. The product is commercially available and consists of micronized hydroxyapatite stabilized on a collagen peptide matrix with a mean molecular weight of 3000-5000 Da and a high safety profile. Both ingredients, hydroxyapatite and collagen peptides, come from fresh bovine bones and are in the form of a homogeneous and dispersible powder. PHC® does not contain any additives, sweeteners, or flavors. No allergies or incompatibilities with drugs, foods or diet supplements have been observed.
DYNAMIC GASTROINTESTINAL DIGESTION
The simulation of human gastrointestinal digestion was carried out using the in vitro Dynamic Digester developed by AINIA (ES 2 361 983 B1). This is a computer-controlled system consisting of interconnected compartments that simulate the gastric and intestinal digestions. The complete process simulates the three digestive steps: oral, gastric, and intestinal digestion. The whole procedure was conducted at a temperature of 37 °C, in darkness and under anaerobic conditions to mimic the physiological conditions of the body, as previously described (25).
To reproduce the oral stage, the process of size reduction (chewing) and enzymatic digestion was simulated. For this purpose, 11.75 g of the sample were dispersed at a temperature of 37 °C in a simulated salivary solution containing α-amylase with a pH between 6.5 and 6.7. This mixture was kept under agitation for 2 min and at a pH between 6.5 and 6.7 (26).
For the gastric stage, the oral digesta was introduced into the gastric module, which already contained a stomach residual solution preheated to 37 °C. Subsequently, the simulation of gastric secretion with pepsin was carried out. During this process, the pH curve was controlled by the addition of 1-M HCl. Thus, the pH of the gastric digesta (mixture of the residual stomach solution and the buccal digesta of the sample) reached a value close to pH 2. The simulation of the transit from stomach to intestine was done continuously according to the Elashoff equation, with a t1/2 of 70 minutes and a factor β equal to 2 (27). The total duration of the gastric digestion process was 3 h.
The subsequent gastric emptyings were mixed with the intestinal media (containing residual solution preheated to 37 °C at the beginning of the process). A simulated intestinal solution containing pancreatin, electrolyte solution, and bile salts was gradually added and maintained at pH 6-6.5 by the addition of 1-M NaHCO3, generating the intestinal digestion media. The intestinal emptying was simulated based on human in vivo data using the Elashoff equation, with a t1/2 of 160 minutes and a factor β equivalent to 1.6. Total transit time at this stage was 6 h (27). Finally, the subsequent intestinal emptyings were accumulated, generating the digested fractions.
CELLS
To carry out intestinal transport studies, the human epithelial cell line Caco-2, obtained from the American Type Culture Collection (ATCC® HTB-37™, USA) was used. Cells were maintained in growth medium containing Eagle Minimum Essential Medium (EMEM) supplemented with 20 % fetal bovine serum (FBS) and antibiotics (penicillin, 100 U/mL; streptomycin, 30 μg/mL), following ATCC recommendations. A biocompatibility test was carried out with the samples to determine the maximum biocompatible concentration of the digested sample with the cell model.
To carry out osteogenic modulation, human osteoblasts (Hob) from bones were used (PromoCell, Heidelberg, Germany). Cells were maintained following PromoCell recommendations and culture media, including Osteoblast Growth Medium and Osteoblast Mineralization Medium. All cell cultures were grown at 37 °C and 5 % CO2.
CACO-2 CELL VIABILITY ASSAY WITH DIGESTED FRACTIONS
The samples used were the soluble fractions generated from the simulated gastrointestinal digestion of PHC®. Also, a blank adding water instead of the sample was generated as control digest. These soluble fractions were thermally treated at 100 °C for 10 min, filtered and frozen until used. The two replicates of each product obtained in the bioaccessibility studies were integrated to perform the cellular assays. A cytotoxicity assay was done using serial dilutions of the samples with Caco-2. For that, the AlamarBlue Cell Viability Reagent (Thermo Fisher, Waltham, MA, USA) was used. After the exposure period (120 min), the reagent was added according to manufacturer instructions and the fluorescence read. Dimethyl sulfoxide (DMSO) at 10 % was used as positive cytotoxic control. The percentage of cell viability relative to control cells (untreated) was determined as follows:
Cell viability (%) = (Fluorescence Units from the sample / Fluorescence Units from the control) x 100
INTESTINAL TRANSPORT STUDIES WITH DIGESTED FRACTIONS
After optimizing culture conditions, intestinal absorption through intestinal epithelial cells was assessed. Briefly, the Caco-2 cells were seeded into polyester membrane (0.4 μm of pore size) inserts and cells were incubated at 37 °C for 21 days for differentiation (28). The integrity of the cell monolayers was determined by measuring transepithelial electrical resistance (TEER) before and after the treatment. Incubation time was 120 min, since it is the contact time in other studies to see collagen effects using this intestinal cell model (17,29). After incubation with digested PHC® at 1/4 dilution (approx. 3 mg/mL of PHC) and control, the basal medium was collected for further treatments of osteoblasts. Only cell monolayers with TEER values higher than 300 Ω cm2 were considered.
PRE-OSTEOBLAST PROLIFERATION WITH INTESTINAL ABSORBED FRACTIONS
For the proliferation experiments, the cells were seeded in multi-well plates in their growth medium, without inducing differentiation. Then, after 24 h in contact with the absorbed fractions, a determination of cell proliferation was made with AlamarBlue® reagent, following manufacturer´s instructions.
OSTEOBLAST DIFFERENTIATION WITH INTESTINAL ABSORBED FRACTIONS
For the differentiation experiments, the protocols recommended by PromoCell were used. For this, the cells were seeded in plates coated with collagen and treated with the differentiation medium, changing the medium every 2-3 days. At 5 or 14 days, cells were in contact with the absorbed fractions obtained after the intestinal transport assay for 24 h, and the cells were collected for their RNA extraction and subsequent analysis of gene expression.
RNA ISOLATION AND QUANTITATIVE REAL-TIME POLYMERASE CHAIN REACTION (QRT-PCR)
After the different cell treatments, cellular RNA was isolated and purified using the MAXWELL equipment (Promega, Madison, WI, USA). RNA quantity and quality was measured by Nanodrop (Thermo Fisher Scientific). Then, the cDNA was obtained using the High-Capacity cDNA reverse transcription kit (Applied Biosystems, Foster City, CA, USA). Real-time PCR was performed with TAQMAN™ fast advanced master mix (Thermo Fisher Scientific) from the cDNA using commercial primers of the selected biomarkers: Runx2 (Hs01047973), collagen 1 (ColI, Hs00164004), alkaline phosphatase (ALP, Hs01029144), and osteocalcin (OC, Hs01587814). All primers were from Thermo Fisher Scientific (Waltham, MA, USA). Also, as housekeeping gene β-actin was used (4326315E). The amplification conditions in the thermocycler (7600 Applied Biosystem) were universal and the quantification of gene expression is carried out in a relative way, so that the magnitude of the physiological changes in the biomarker gene is obtained in comparison with a reference gene.
STATISTICAL ANALYSES
Results are presented as mean ± standard error of the mean (SEM) of at least two independent studies and 2-3 replicates. Statistical significance between different conditions (treated with PHC® vs digested control) was assessed using Student´s t-test with Welch´s correction applied in case of significantly different variances (F-test). The tests were performed using the GraphPad Prism software 8.3.1. A value of p ≤ 0.05 was considered significant.
RESULTS
ASSESSMENT OF CACO-2 CELL BIOCOMPATIBILITY
Epithelial intestinal cells were in contact with serial dilutions of digested fractions and its cell viability was measured. Results showed that using a 1/4 dilution of both digested control and digested PHC® fraction (approx. 3 mg/mL) no toxic effects were observed, since it was the first dilution that showed no differences with the untreated control. At 1/2 dilution (approx. 6 mg/mL), digested PHC® fraction exhibited biocompatibility unlike the digestion control sample (Fig. 2). The positive control DMSO showed cytotoxic effects, as expected.

Figure 2. Cell viability of intestinal epithelial cells (Caco-2) after 2 h in contact with digested Phoscollagen® and control fractions (DMSO: dimethyl sulfoxide; n = 3)
For all subsequent studies, the absorbed PHC® fraction obtained from 3 mg/mL of digested PHC® and its equivalent control were used.
ASSESSMENT OF PRE-OSTEOBLAST PROLIFERATION
Pre-osteoblasts were in contact with the absorbed fractions and changes in cell proliferation were measured. After 24 h of treatment, pre-osteoblasts showed a significant proliferation in contact with the absorbed PHC® fraction compared to the absorbed control (Fig. 3).
ASSESSMENT OF OSTEGENIC CAPACITY
The mRNA expression of osteogenic biomarkers, including Runx2, ALP, ColA1 and OCN, were evaluated in primary osteoblasts previously differentiated for 5 days or 14 days.
Compared to the control, 24 h of treatment with the absorbed PHC® fraction caused no changes in osteogenic biomarkers in osteoblasts with 5 days of differentiation (Fig. 4A) but, with 14 days of differentiation (Fig. 4B), -x- an increase in all gene biomarkers was detected, being statistically significant in Runx2 (p = 0.0008), ColA1 (p = 0.035) and OCN (p = 0.027).
DISCUSSION
This study confirms osteogenic effects of a hydrolyzed collagen-hydroxyapatite complex (Phoscollagen®, PHC®) by proliferation and differentiation of osteoblasts, the bone cells that synthesize bone extracellular matrix and regulate its mineralization. In fact, osteoblasts carry out the anabolic processes involved in bone formation, during which they mature and express specific genes necessary for its achievement.
Bioavailable PHC® fractions derived from simulated gastrointestinal digestion and absorption induce human osteoblasts to notoriously increase mRNA expression of the following osteogenic biomarkers measured by real-time PCR: 1) COL1A1 (p ≤ 0.05), the gene that encodes α1 chains of type-1 pro-collagen, essential for collagen synthesis and bone formation; 2) Runx2 (p ≤ 0.001), the specific transcription factor for osteogenesis, determinant for osteoblastic lineage differentiation from mesenchymal cells under stimulation of specific genes (COL1A1, ALP and OC); 3) ALP, an enzyme that provides inorganic phosphate, which binds to calcium, regulating mineralization (in this case, the increase does not reach significance); 4) osteocalcin (OC, p ≤ 0.05), the most abundant non-collagenous protein in the extracellular matrix, synthesized by osteoblasts; it acts as a hormone, elevating bone category to endocrine organ.
It is noteworthy that OC is involved in glucose homeostasis, skeletal muscle function, brain development and male fertility. Also, it has a preventive effect on hepatic steatosis and arterial calcification (30). This suggests that stimulating bone formation and, in particular, secretion of OC, may have therapeutic implications beyond bone structure good condition and could be one of the mechanisms involved in the lipid and glucose modulating effects detected in collagen peptides (31-33).
The results obtained in this study about cell growth and gene expression are consistent with those obtained in other studies that have detected osteogenic activity produced either by collagen peptides (16,34-36) or by combinations of these with calcium and phosphorus (37).
Unlike those studies, the present study carries out a simulation of gastrointestinal digestion and absorption, which implies a greater approximation to in vivo systems and allows to include the influence in osteogenic activity not only of the absorbed collagen peptides, calcium and phosphorus, but also of the metabolites generated by Caco-2 cells when interacting with the digested PHC® fraction. Furthermore, it has been observed that the biocompatibility of the digested PHC® fraction (at 1/2 dilution, aprox. 6 mg/mL) with Caco-2 cells is significantly higher than that of digested control fraction, showing a protective effect on intestinal epithelial cells which has also been detected in collagen peptides (38).
Other studies on collagen peptides have carried out different in vivo approaches with success either using human serum after ingesting hydrolyzed collagen to treat osteoblast and osteoclast cell cultures (39) or combining in vitro and in vivo trials in which beneficial effects on bone mass and microarchitecture have been correlated with an increase in osteoblast proliferation, gene expression, and collagen synthesis (17-31,40).
Among the studies cited above, special mention should be made of Wu's, 2020 (37), which evaluated how the introduction of a phosphate group (phosphorylation) and calcium chelation of collagen peptides affected their osteogenic activity and ability to improve calcium absorption. Thus, the effects of 4 groups of compounds were studied: collagen peptides (CP), phosphorylated collagen peptides (PCP), calcium-chelated collagen peptides (CP-Ca), phosphorylated calcium-chelated collagen peptides (PCP-Ca). All groups significantly increased the proliferation of mouse pre-osteoblasts, as well as the mRNA expression levels of osteoblast differentiation biomarkers and mineralization. However, the most osteogenically active group was PCP-Ca, the one with a composition most closely related to the hydrolyzed collagen-hydroxyapatite complex evaluated in this study. This fact suggests a higher anabolic efficacy when collagen peptides are adequately bound with calcium and phosphorus compounds than when they are alone, possibly due in part to synergy between funcional and nutricional actions of collagen peptides and specific inorganic nutrients closely related to bone tissue composition.
In conclusion, this study shows the osteogenic activity of a hydrolyzed collagen-hydroxyapatite complex (Phoscollagen®) after simulating its digestion and absorption using in vitro methods, based on its effect on preosteoblast proliferation and on gene biomarker mRNA expressions as involved in the bone formation process.
Furthermore, taking into account current knowledge and evidence on the nutritional strategy to prevent and assist bone loss pathology and fractures, the protein-mineral complex under study brings together several nutritional aspects that can enhace bone formation from a diet supplementation approach: 1) bioactivity of collagen peptides, which promotes bone metabolism more effectively than non-hydrolyzed and/or non-collagenic proteins; 2) binding capacity of collagen peptides to increase calcium absorption; 3) physiological Ca/P ratio supported by hydroxyapatite, which prevents decalcification and allows mineralization; 4) nutrition of both organic and mineral main bone components, using the food compounds most naturally related to bone tissue composition; 5) synergy between nutritional and functional action, gathered in a homogeneous food supplement aimed to be easily incorporated into the diet.