Mi SciELO
Servicios Personalizados
Revista
Articulo
Indicadores
-
Citado por SciELO
-
Accesos
Links relacionados
-
Citado por Google
-
Similares en SciELO
-
Similares en Google
Compartir
Revista de Osteoporosis y Metabolismo Mineral
versión On-line ISSN 2173-2345versión impresa ISSN 1889-836X
Rev Osteoporos Metab Miner vol.9 no.1 Madrid ene./mar. 2017
https://dx.doi.org/10.4321/s1889-836x2017000100002
Originales
Vascular endothelial growth factor (VEGF) and the N-terminal portion of parathyroid hormone-related protein (PTHrP) regulate the proliferation of human mesenchymal stem cells
1Facultad de Medicina. Instituto de Medicina Molecular Aplicada (IMMA). Universidad CEU San Pablo. Madrid (España)
2Facultad de Medicina. Instituto de Medicina Molecular Aplicada (IMMA). Universidad CEU San Pablo. Madrid (España)
3Facultad de Medicina. Instituto de Medicina Molecular Aplicada (IMMA). Universidad CEU San Pablo. Madrid (España)
4Fundación HM Hospitales. Madrid (España)
5Facultad de Medicina. Instituto de Medicina Molecular Aplicada (IMMA). Universidad CEU San Pablo. Madrid (España)
Introduction
In the late 1960s, Friedenstein first described Bone Marrow Stromal Cells (BMSCs) as fibroblastic cells with adhesion to the plastic and tri-linear differentiation capacity, generating osteoblasts, chondroblasts and adipocytes. A fraction of these cells also demonstrated clonogenic capacity when they were cultured in very low density (Colony Forming Units-Fibroblast, CFU-F) [1]. It was later noted that this multipotentiality is only inherent in a small part of this heterogeneous cell population, corresponding to Skeletal Stem Cells (SSCs, less than 0.1% of BMSCs and about 12% of CFU-F), which are also the only ones able to produce the stromal necessary for generating hematopoietic niches in bone marrow [2,3].
Adipose tissue contains a large number of adherent cells, capable of forming CFU-F and many other features that liken them to BMSCs. Compared to BMSCs, this tissue is easily accessible, such as liposuction discarded in liposuction operations, and isolation of stromal cells is relatively simple [4,5]. Similar to the SSCs among BMSCs, the stromal vascular fraction (SVF) of adipose tissue is a heterogeneous population of cells, that include the ASCs, which presumably are similarly associated with SSCs to the microvasculature of fat [6], in consonance with other tissues in which the adult stem cells are associated with the microvasculature in the form of pericytes [7]. Although several authors claim that ASCs have the inherent ability to differentiate into bone-derived cells, to date no research group has demonstrated that this is possible except after transdifferentiating these cells after intensive treatment with BMPs, which has been shown to be a potent osteo-inductor of various cell types [8]. Although the clinical utility of ASCs for bone regeneration has not been demonstrated, these cells may be a convenient model for studying osteoblastic differentiation in vitro, because of its easy access and response similar to that of BMSCs to in vitro tri-linear differentiation factors [9].
In the presence of ascorbic acid, dexamethasone and β-glycerol-phosphate the ASCs express markers of osteoblastic differentiation in vitro. This is the case of the system consisting of osteoprotegerin (OPG) and activator receptor ligand for the nuclear factor kB (RANKL), proteins involved in bone remodeling. RANKL is a protein of the tumor necrosis factor α (TNF-α) family that is expressed on the membrane of osteoblasts, which in turn can be secreted by them [10]. It binds to the activator receptor for the nuclear factor kB (RANK) that is present in osteoclast precursors activating its differentiation and maturation to osteoclasts [11]. OPG is a decoy receptor secreted by osteoblasts that binds to RANKL, preventing it from binding to RANK, thereby blocking the activation of osteoclasts. On the other hand, Runx2 is a key transcription factor in the differentiation of osteoblastic cells [12].
Vascular endothelial growth factor (VEGF) is a key molecule in the regulation of endothelial cell proliferation. It promotes the proliferation, migration and survival of these cells, as well as their vascular permeability [13]. The expression of VEGF and its receptors in BMSCs has been demonstrated by various studies in cell cultures [13,14]. In addition, the role of the VEGFR2 receptor as key in osteoblastic differentiation and survival has been shown in vitro [15]. VEGF induces differentiation in cell cultures of preosteoblasts [14] and stimulates their migration and proliferation [13,14]. Thus, VEGF seems to be involved in the early stages of bone differentiation, both in skeletogenesis –an important factor in endochondral and intramembranous bone formation– and in adult homeostasis, promoting osteoblastic differentiation and reducing adipogenic differentiation of BMSCs [16,17].
Parathormone-related protein (PTH), PTHrP, is a pleiotropic cytokine with important functions in bone tissue [15]. It is considered a modulator of bone remodeling and a stimulator of bone formation that promotes osteoblastic differentiation and its survival [15]. It is, therefore, an important maturation factor. The post-translational processing of the PTHrP gene generates different fragments, including an N-terminal fragment, PTHrP (1-36), which shows great homology with PTH and acts through the common 1 receptor for PTH/PTHrP, PTH1R [12]. The expression of PTHrP in the osteoblastic lineage decreases as it differentiates [20], but PTH1R plays a fundamental role in mature osteoblasts and osteocytes, decreasing its apoptosis and increasing its number in periosteum osteoblasts in vivo [18,19]. Previous studies indicate that PTHrP could increase the proliferation of immature osteoblasts through the regulation of Cyclin D1 (promoter) and p27 (inhibitor), both regulators of the cell cycle [12].
In this paper, we hypothesize that the effects of VEGF and PTHrP on cell proliferation of osteoprogenitors are dependent on the osteoblastic differentiation stage. Thus, more undifferentiated populations would be more sensitive to VEGF while the progenitors already committed to osteoblastic differentiation would respond better to PTHrP (1-36). For this purpose, human ASCs from liposuction were used as an in vitro model of osteogenic differentiation. After confirming the model’s in vitro differentiation ability by Runx2 gene expression and calcium accumulation, the proliferative response to stimuli with VEGF or PTHrP (1-36) of ASCs subjected to or not undergoing osteogenic induction was analyzed. VEGF, but not PTHrP (1-36), induced proliferative capacity of undifferentiated ASCs, whereas PTHrP (1-36), but not VEGF, induced proliferation of ASCs previously treated with osteogenic differentiation medium, confirming the differential role of these growth factors in different stages of differentiation.
Materials and methods
Isolation, primary culture and functional study of ASCs
Abdominal subcutaneous adipose tissue was obtained during the surgery of healthy patients using the liposuction technique. Six women were included in this study with an average age of 40 years (range 25-60 years). All donors gave their informed consent, according to the appropriate clinical protocol. Patients were operated in the Department of Plastic Surgery of HM Hospitals (Madrid, Spain), and tissue sample collection was approved by the Institutional Review Board/Clinical Research Ethics Committee of HM Hospitals (Madrid, Spain). Adipose tissue was digested in 0.075% collagenase solution type I (Invitrogen, Life Technologies, New York, USA) for 30 minutes at 37°C, following the protocol described previously [4]. The cells were then plated on plastic surface (Corning, New York, USA) for 24 hours in DMAX Growth Medium (Invitrogen, Life Technologies, New York, USA) with 10% FBS (Sigma St. Louis, Missouri, USA) and supplemented with antibiotics: penicillin (100 IU/ml) and streptomycin (100 mg/ml) (Sigma-Aldrich, St. Louis, Missouri, USA). Non-adherent cells were removed, and fresh medium was added for the primary culture of the adherent cell fraction for 7 days. The culture medium was replaced every 3 days. At that time, ASCs were functionally phenotyped by flow cytometry and their potential for osteogenic differentiation was analyzed.
Flow cytometry
For flow cytometry analysis, ASCs were re-suspended in PBS (saline phosphate buffer) at a density of 1x10 6 cells/ml, fixed with 2% paraformaldehyde (PFA) and incubated with conjugated mouse monoclonal antibodies (FITC or PE) with anti-CD90, CD29, CD34, CD45, CD106 CD44, CD144, CD31 and KDR (BioLegend, San Diego, CA, USA) for 30 min at room temperature. Cells were washed 3 times with PBS and analyzed by Accuri's C6 Flow System cytometer at 488 nm and 15 mW. Frontal dispersion (FSC), lateral dispersion (SSC) and specific fluorescent marker (LGFL) at 488 nm and 540 nm were automatically obtained for each cell. Data were digitally collected over a dynamic range of 16 million digital data channels. Amplification and logarithmic analysis of fluorescence was performed using BD Accuri™ c6 Analysis Software.
Assays of osteogenic differentiation
Cell lineage osteogenic differentiation medium was used to evaluate the potential for osteogenic differentiation of ASC. For this differentiation, ASCs were cultured for 14 days in the presence of DMEM (Dulbecco's Modified Eagle medium) with 10% FBS, 10-8 M dexamethasone, 0.5 mg/ml ascorbic acid and 0.1 M b-glycerolphosphate Sigma-Aldrich, St. Louis, Missouri, USA). The medium was replaced every 3 days, and at the end of the 14-day period, the histochemical red alizarin staining was performed to reveal and quantify the number of cells per microscopic field surrounded by mineralized extracellular matrix stained with alizarin red. A check of osteoblastic differentiation was also performed by means of alkaline phosphatase (ALP) assay, which consisted of the identification of the red stained deposits indicating alkaline phosphatase activity by the Sigma Aldrich 86R kit. ASCs from six different patients were used in each test.
Cellular proliferation studies
The response related to the proliferation of ASCs to factors such as VEGF (160 pM) and PTHrP (1-36) (100 nM) in the cultures were studied for 24 hours in both growth medium and osteogenic differentiation medium. Measuring the proliferation of these assays, the xCelligent System (Roche Diagnostics, Basel, Switzerland) was used to measure cell proliferation in real time. The xCelligent system provided real-time and end-point proliferation measurements based on readings of cultured plates with electrodes that detected changes in cell morphology, providing a parameter called Cell Index extrapolable to cell proliferation.
Extraction of total RNA and quantitative PCR in real time
ASCs, both at baseline and at differentiated conditions to osteoblasts, were subjected to extraction of total RNA from a cell homogenate with a standard method with guanidyl-phenol-chloroform thiocyanate (Tri-Reagent, MCR; Cincinnati, Ohio, USA). The purity and quantification of total RNA extracted was determined by spectrophotometry A260/A280 (Nanodrop 2000/2000c Spectrophotometer, Thermo Scientific). Synthesis of cDNA was performed using random oligonucleotides and a reverse transcriptase (High capacity RNA to cDNA Kit, Applied Byosystem; Foster City, Calif., USA). Gene expression analysis by real-time RT-PCR was performed with resulting cDNA, using a heat-activated polymerase, TaqDNA (Taqman gene expression master mix, Applied Byosystem, Foster City, California, USA) and human-specific primers For RUNX2, (Hs00231692 m1) OPG (TNFRSF1 Hs 00171068), VEGF-A (Hs 00173626 m1) and VEGFR2 (Hs 00176676 m1) (Applied Byosystem; Foster City, California, USA). After an initial incubation of 10 minutes at 25°C and another of 2 hours at 37°C, the samples were cycled at 4°C. The results were expressed as expression levels of each gene (once normalized to the 18s RNA as constitutive gene) in each experimental condition, relative to its corresponding control: 2 -ΔCt, where Ct represents the PCR threshold cycle in the Which the program detects for the first time an appreciable increase of fluorescence on the basal signal. All determinations were performed in duplicate. (ΔCt = Ct (gene of interest) - Ct (18S endogenous control).
Statistical analysis
Results were expressed as mean +/- Standard Deviation (SD). The non-parametric comparison between the means of two samples was performed by the Mann Whitney test. Non-parametric ANOVA was used to compare several samples (Kruskal-Wallis). All values with p <0.05 were considered significant.
Results
Firstly, the characterization of ASCs from the primary culture of liposuction was carried out. Phenotypic analysis of ASC by flow cytometry revealed that 99.6% were CD90+, 91,6% CD44+, 90,4% CD 29+, 4,2% CD34+, 2,2% CD45+. In addition, the markers CD106, CD155, KDR and CD31, all negative for ASCs (Table 1), were analyzed.
Table 1 Characterization by flow cytometry of ASCs isolated from adipose tissue obtained by liposuction

To evaluate the potential for osteogenic differentiation of ASC, they were subjected to osteoblastic differentiation in vitro. As shown in Figure 1A, ALP activity revealed that ASCs in osteogenic differentiation medium had undergone such differentiation. The red cytochemical staining of alizarin (Figure 1B) also revealed the positive result of osteogenic differentiation.
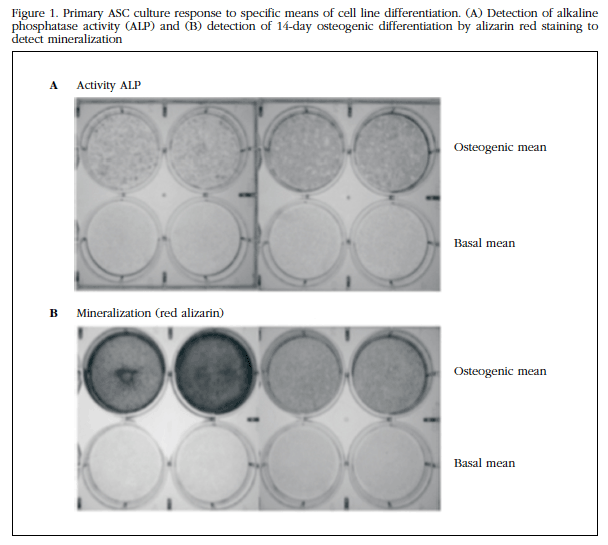
Figure 1 Primary ASC culture response to specific means of cell line differentiation.(A) Detection of alkaline phosphatase activity (ALP) and (B) detection of 14-day osteogenic differentiation by alizarin red staining to detect mineralization
Gene expression was evaluated for some of the markers of osteogenic differentiation such as OPG and Runx2 [12,13,24]. The expression of these two markers was significantly increased (p <0.05) after 14 days of culture with the osteogenic differentiation medium (Figure 2A-B).

Figure 2 Changes in gene expression (analyzed by real-time PCR) of bone differentiation factors: (A) OPG, (B) Runx2, at different times of osteoblastic differentiation
The gene expression of the VEGFA system and its receptor induced by osteogenic differentiation was evaluated. As can be seen in Figure 3, after 7 days of differentiation the cells significantly decrease the expression of VEGF and its type 2 receptor.
Cell proliferation assays with media supplemented with PTHrP and VEGF showed different results. A proliferation study of the ASCs in response to VEGF (160 pM) was performed for 24 hours under basal conditions (growth medium) or with osteogenic differentiation medium.

Figure 3 Changes in gene expression after 7 days of differentiation (analyzed by real-time PCR) of angiogenesis factors: (A) VEGF-A and (B) VEGFR2
As shown in Figure 4, VEGF significantly increased the proliferation of ASCs in growth medium, but had no significant effect on proliferation of cultured cells in osteogenic differentiation medium (p <0.05). The results indicated that VEGF increased the proliferation of ASCs, but provided they did not begin their differentiation process. In response to PTHrP (1-36) (100 nM) for 24 hours, ASCs did not significantly alter their proliferation index (Figure 4A). When the above-described proliferation assay was performed on osteogenic differentiation medium, it was found to change the ASC phenotype, and altered its responsiveness to these factors. Thus, in this case, PTHrP increased proliferation index in cells already committed to osteoblastic differentiation (Figure 4B).
Discussion
In the present study, ASCs from healthy women were exposed to short treatments with PTHrP and VEGF under different differentiation conditions and their proliferative capacity was analyzed. Our results indicate that the more undifferentiated populations would be more sensitive to VEGF while the progenitors already committed to osteoblastic differentiation respond better to PTHrP (1-36), showing a greater expression of PTH/PTHrR (PTH1R) receptor 1. Human ASCs derived from liposuction as an in vitro model of osteogenic differentiation were used. During this study the characterization of the ASCs was carried out by a study of the cell surface markers. The data obtained were those expected according to previous studies [21,22]. The capacity of osteoblastic differentiation of ASCs was also studied. Such differentiation was verified by the ALP activity of these cells after 14 days in osteogenic differentiation medium. Similarly, histochemical staining of alizarin red was used to verify such differentiation thereby complementing the analysis of ALP activity. In addition, the gene expression of some markers such as OPG and Runx2, [16,23] was evaluated. Runx2 is essential for osteoblastic differentiation, leading ASCs to the osteoblastic lineage and inhibiting their differentiation into adipogenic or chondrogenic lineage [23]. In addition, Runx2 has been described as a factor that keeps osteoblasts in the immature state without differentiating into osteocytes [23]. Likewise, previous studies consider OPG as a marker of early osteogenic differentiation in human mesenchymal cells [24]. As expected, our data show that osteogenic differentiation induces an increase in the expression of these two markers, OPG and Runx2, with respect to the undifferentiated state.
Gene expression of the VEGFA system and its VEGFR2 receptor were also evaluated under conditions of osteogenic differentiation. After seven days in the presence of the osteogenic differentiation medium, a decrease in the expression of VEGF and its type 2 receptor was observed. In our study, we hypothesized that the effects of VEGF and PTHrP on cell proliferation of osteoprogenitors are dependent on the stage of differentiation Osteoblast. Thus, the more undifferentiated populations would be more sensitive to VEGF due to their proximity to the endothelial niche in vivo, whereas the already compromised progenitors towards osteoblastic differentiation would respond better to PTHrP (1-36) by showing the PTH1R receptor a more important role in osteoblasts Mature and osteocytes. After confirming the model’s in vitro differentiation ability by Runx2 gene expression and mineralization, the proliferative response to VEGF or PTHrP (1-36) stimuli of ASCs subjected to or not undergoing osteogenic induction was analyzed. VEGF, but not PTHrP (1-36), favored the proliferative capacity of uninduced ASCs, whereas PTHrP (1-36), but not VEGF, favored the proliferation of previously differentiated ASCs, confirming the differential role of these growth factors in different stages of differentiation. Previous studies indicate that secreted VEGF is critical in the differentiation of BMSCs into osteoblasts, hindering their differentiation to other cell lineages such as adipogenic [16,17]. Likewise, Alonso et al. [15] have shown how VEGF and PTHrP modulate the differentiation and survival of osteoblasts. Our results from the proliferation study of ASCs in response to VEGF for 24 hours in growth medium or with osteogenic differentiation medium show an increase in the proliferation of ASCs grown in normal medium but without significant effects on cell proliferation cultured in the middle of osteogenic differentiation, supporting the notion that VEGF exerts a preponderant role on the regulation of proliferation in early stages of differentiation, although previous studies also point to an implication of VEGF in the survival of osteoblasts [15].
After 24 hours of treatment with PTHrP (1-36), undifferentiated ASCs did not alter their proliferation index. However, in the presence of osteogenic differentiation medium, PTHrP (1-36) significantly increased proliferation, pointing to the implication of this factor in the proliferation of BMSCs involved in osteogenic differentiation in vivo.
Thus, we may conclude that, although previous studies have shown that VEGF and PTHrP modulate the differentiation and survival of osteoblasts [15], these factors could regulate the proliferation of osteoprogenitors depending on their commitment or not to the osteoblastic differentiation, with VEGF more involved in the proliferation of more undifferentiated progenitors close to the perivascular niche, whereas PTHrP (1-36) would stimulate cells more involved in osteoblastogenesis.
Referencias
1 Friedenstein AY, Lalykina KS. Lymphoid cell populations are competent systems for induced osteogenesis. Calcif Tissue Res. 1970;Suppl:105-6. [ Links ]
2 Bianco P, Robey PG, Simmons PJ. Mesenchymal stem cells: revisiting history, concepts, and assays. Cell Stem Cell. 2008;2(4):313-9. [ Links ]
3 Sworder BJ, Yoshizawa S, Mishra PJ, Cherman N, Kuznetsov SA, Merlino G, et al. Molecular profile of clonal strains of human skeletal stem/progenitor cells with different potencies. Stem Cell Res. 2015;14:297-306. [ Links ]
4 Van Harmelen V, Röhrig K, Hauner H. Comparison of proliferation and differentiation capacity of human adipocyte precursor cells from the omental and subcutaneous adipose tissue depot of obese subjects. Metabolism. 2004;53:632-7. [ Links ]
5 Prunet-Marcassus B, Cousin B, Caton D, André M, Pénicaud L, Casteilla L. From heterogeneity to plasticity in adipose tissues: site-specific differences. Exp Cell Res. 2006;312:727-36. [ Links ]
6 Su X, Lyu Y, Wang W, Zhang Y, Li D, Wei S, et al. Fascia Origin of Adipose Cells. Stem Cells. 2016; 34:1407-19. [ Links ]
7 Nakagomi T, Kubo S, Nakano-Doi A, Sakuma R, Lu S, Narita A, et al. Brain vascular pericytes following ischemia have multipotential stem cell activity to differentiate into neural and vascular lineage cells. Stem Cells. 2015;33:1962-74. [ Links ]
8 Bianco P, Robey PG. Skeletal stem cells. Development. 2015;142:1023-7. [ Links ]
9 Gimble JM, Katz AJ, Bunnell BA. Adipose-derived stem cells for regenerative medicine. Circ Res. 2007;100: 1249-60. [ Links ]
10 Khosla S. Minireview: the OPG/RANKL/RANK system. Endocrinology. 2001;142:5050-5. [ Links ]
11 Cowan CM, Shi YY, Aalami OO, Chou YF, Mari C, Thomas R, et al. Adipose-derived adult stromal cells heal critical-size mouse calvarial defects. Nat Biotechnol. 2004;22:560-7. [ Links ]
12 Esbrit P, Herrera S, Portal-Núñez S, Nogués X, Díez-Pérez A. Parathyroid Hormone-Related Protein Analogs as Osteoporosis. Calcif Tissue Int. 2016;98: 359-69. [ Links ]
13 Ferrara N, Gerber HP, LeCouter J. The biology of VEGF and its receptors. Nat Med. 2003;9:669-76. [ Links ]
14 Deckers MM, Karperien M, van der Bent C, Yamashita T, Papapoulos SE, Löwik CW. Expression of vascular endothelial growth factors and their receptors during osteoblast differentiation. Endocrinology. 2000;141: 1667-74. [ Links ]
15 Alonso V, Gortázar AR, Ardura JA, Andrade-Zapata I, Alvarez Arroyo MV, Esbrit P. Parathyroid homone-related protein (107-139) increases human osteoblastic celle survival by activation os vascular endothelial growth factor receptor-2. J Cell Physiol. 2008;217:717-27. [ Links ]
16 Liu Y, Olsen BR. Distinct VEGF functions during bone development and homeostasis. Arch Immunol Ther Exp (Warsz). 2014;62:363-8. [ Links ]
17 Liu Y, Berendsen AD, Jia S, Lotinun S, Baron R, Ferrara N, et al. Intracellular VEGF regulates the balance between osteoblast and adipocyte differentiation. J Clin Invest. 2012;122:3101-13. [ Links ]
18 Jilka RL, Weinstein RS, Bellido T, Roberson P, Parfitt AM, Manolagas SC. Increased bone formation by prevention of osteoblast apoptosis with parathyroid hormone. J Clin Invest. 1999;104:439-46. [ Links ]
19 Jilka RL, O'Brien CA, Ali AA, Roberson PK, Weinstein RS, Manolagas SC. Intermittent PTH stimulates periosteal bone formation by actions on post-mitotic preosteoblasts. Bone. 2009;44:275-86. [ Links ]
20 Suda N, Gillespie MT, Traianedes K, Zhou H, Ho PW, Hards DK, et al. Expression of parathyroid hormone-related protein in cells of osteoblast lineage. J Cell Physiol. 1996;166:94-104. [ Links ]
21 Gimble J, Guilak F. Adipose-derived adult stem cells: isolation, characterization, and differentiation potential. Cytotherapy. 2003,5:362-9. [ Links ]
22 Dominici M, Le Blanc K, Mueller I, Slaper-Cortenbach I, Marini F, Krause D, et al. Minimal criteria for defining multipotent mesenchymal stromal cells; The International Society for Cellular Therapy position statement. Cytotherapy. 2006;8:315-7. [ Links ]
23 Komori T. Regulation of osteoblast differentiation by transcription factors. J Cell Biochem. 2006;99(5):1233-9. [ Links ]
24 Song SJ, Jeon O, Yang HS, Han DK, Kim BS. Effects of culture conditions on osteogenic differentiation in human mesenchymal stem cells. J Microbiol Biotechnol. 2007;17:1113-9. [ Links ]
Received: September 16, 2016; Accepted: December 20, 2016