Mi SciELO
Servicios Personalizados
Revista
Articulo
Indicadores
-
Citado por SciELO
-
Accesos
Links relacionados
-
Citado por Google
-
Similares en SciELO
-
Similares en Google
Compartir
Revista de Osteoporosis y Metabolismo Mineral
versión On-line ISSN 2173-2345versión impresa ISSN 1889-836X
Rev Osteoporos Metab Miner vol.11 no.4 Madrid nov./dic. 2019 Epub 06-Abr-2020
https://dx.doi.org/10.4321/s1889-836x2019000400005
ORIGINALS
Factors that influence the results of bone ultra-microindentation tests. An experimental study in rats
1Laboratory of the Material Science and Engineering Division (LADICIM). Higher Technical School of Civil Engineers, Channels and Ports. University of Cantabria. Santander (Spain).
2Traumatology and Orthopedics Service. Marqués de Valdecilla University Hospital. Department of Medical and Surgical Sciences. Marqués de Valdecilla Research Institute (IDIVAL). University of Cantabria. Santander (Spain).
3Core MKT Consulting. Ciudad Real (Spain).
4Department of Internal Medicine. Marqués de Valdecilla University Hospital. Marqués de Valdecilla Research Institute (IDIVAL). University of Cantabria. Santander (Spain).
Objetive:
The properties of the materials that constitute the bone tissue are decisive in its mechanical strength but the factors that influence it are partially unknown at present.
Material and method:
In this paper, we gauge bone hardness by means of ultra-microindentation tests with a Berkovich tip and a 150 mN load in femurs of Sprague-Dawley rats subjected to a transverse fracture or a subtraction osteotomy. The results are compared in different bone locations and experimental groups. The study includes the following four experimental groups, each consisting of four rats: a) standard diaphyseal fracture; b) fracture plus osteotomy of 2 mm; c) osteotomy treated with human parathyroid hormone, PTH (1-84); d) osteotomy treated with strontium ranelate.
Results:
We found the hardness of the material was consistently greater in cortical bone than in trabecular bone. It was also consistently higher in the upper femoral epiphyses than in the lower epiphyses (difference of 1.2 standard deviations). The surgery reduced hardness in the operated femur (difference of 0.3 standard deviations, p=5.5 x10-2). PTH treatment induced a slight but consistent increase in hardness at all sites (p=1.8x10-5) while the effect of strontium ranelate was inconsistent.
Conclusions:
These data show that tissue micro-hardness is influenced by a variety of factors, including anatomy, type of bone tissue, skeletal injury and drug therapy. Therefore, future studies on tissue quality should be carefully designed with these factors in mind.
Key words: bone quality; ultra-microindentation; bone hardness
INTRODUCTION
Fragility fractures are the relevant hallmark of osteoporosis1. The risk of fracture is closely related to bone strength, which, in turn, depends on bone mass, geometry and material quality2 3 4 5-6. Bone mass and geometry can be evaluated clinically using bone densitometry and high resolution imaging techniques. However, the mechanical properties of bone tissue are more difficult to explore. These properties determine bone quality, a concept that represents the intrinsic capacity of tissue to resist tension states, regardless of the amount of material (bone density) or its spatial distribution (bone architecture). Bone quality depends on the chemical composition and organization of the bone matrix7.
In an indentation or hardness test, a sample is subjected to quasi-static loading by means of a small indenter, recording the size of the resulting footprint; Sometimes the curve that relates the applied load and the displacement experienced by the indenter during the test is also determined. Hardness is defined as the maximum force applied divided by the area of the footprint that remains in the material after the test. Hardness is the property of the material that characterizes its resistance to permanent/plastic deformation8.
Ultra-microindentation (UMI) allows hardness tests to be carried out on the trabecular scale, on individual trabeculae and bone osteons. Several pre-clinical models suggest that the results may be a marker of skeletal resistance. The main advantages of UMI tests are the simplicity of the technique and the ability to map microhardness in different areas of a sample8. However, the factors that influence bone tissue hardness results are only partially known, which limits the possibility of carrying out comparisons between studies. This is a relevant aspect, particularly in view of the recent introduction of the ultra-microindentation technique in humans9. In this sense, the objective of this study was to explore the variability of hardness in different skeletal locations, as well as the changes induced by various interventions in an experimental model.
MATERIAL AND METHODS
Study group.Sprague-Dawley rats (13 weeks old) had been employed as part of a study of delayed consolidation of femoral fractures, using a retrograde intramedullary screw inserted through the intercondylar region of the knee for fixation. Details have been published previously10. Study groups (4 rats each) included: a) transverse diaphyseal fracture; b) fracture plus 2 mm diaphyseal subtraction osteotomy (SO); c) SO treated with human parathyroid hormone, PTH (1-84) (30 mcg/kg/day subcutaneously); d) SO treated with strontium ranelate (SR) (900 mg/kg/day orally). Twelve weeks after surgery, the animals were sacrificed, both femurs were removed and stored at -18ºC until analyzed.
Hardness tests.The upper and lower epiphyses of the non-operated femurs, as well as the upper epiphysis of the operated femurs, were carefully sectioned and embedded in acrylic resin. The lower epiphyses of the operated femurs could not be analyzed due to alterations induced by screw insertion. The cross sections were polished with silicon carbide paper and subsequently with aluminum oxide with a particle size decreasing to 0.05 mm. Before the test, the samples were immersed in a calcium phosphate buffer solution at 37ºC, to mimic the physiological conditions. Hardness was analyzed at 1215 points randomly selected from the cortical and trabecular regions, using a DUH 211 ultra microindenter test (Shimadzu) with a diamond-made Berkovich tip. The test parameters were as follows: loading speed, 2,665 mN/s; maximum load (Pmax), 150 mN; load maintenance time, 10 s; download speed, 2,665 mN/s. After discharge, the residual footprint area (Ar) was measured with an optical microscope and the hardness of the material was estimated as Pmax/Ar.
Data analysis.The study data were distributed in groups according to the independent variables (trabecular or cortical tissue, upper or lower epiphysis, operated or non-operated femur, type of surgery and drug therapy). The UMI data of each group were subjected to a goodness test of fit X2to confirm that they were homogeneous and that they followed a normal distribution. Only groups with p>0.95 were considered usable for the purposes of the present analysis. An unpaired two-tailed t-test was used for pairwise comparisons and a p-value with Bonferroni correction was calculated. Next, the overall difference between sets of samples that were similar was estimated except for a single distinctive predictor variable to assess their influence. For this, the Hedge g was calculated, which is equivalent to the difference between groups expressed in Zscore. Random effects models were used for these calculations, implemented in the Meta-Essentials program (http://www.erim.eur.nl/research-facilities/meta-essentials).
RESULTS
Table 1summarizes the conditions and the results obtained (the mean and standard deviation of hardness) for each of the 32 experimental groups. The last column ('Analyzable') indicates the result of the goodness test of adjustment X2; it should be taken into account that only four groups were not analyzed. The g values of the comparisons between groups are represented in figure 1.
Table 1. Experimental groups and hardness values
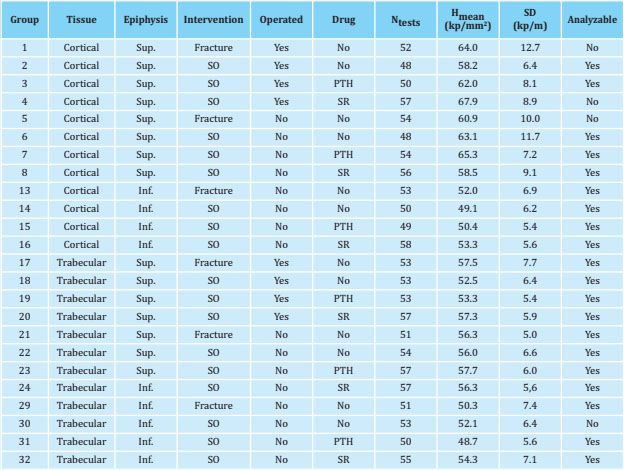
Hmean: average; SD: standard deviation; Sup: superior; Inf: inferior; SR: stroncio ranelate.
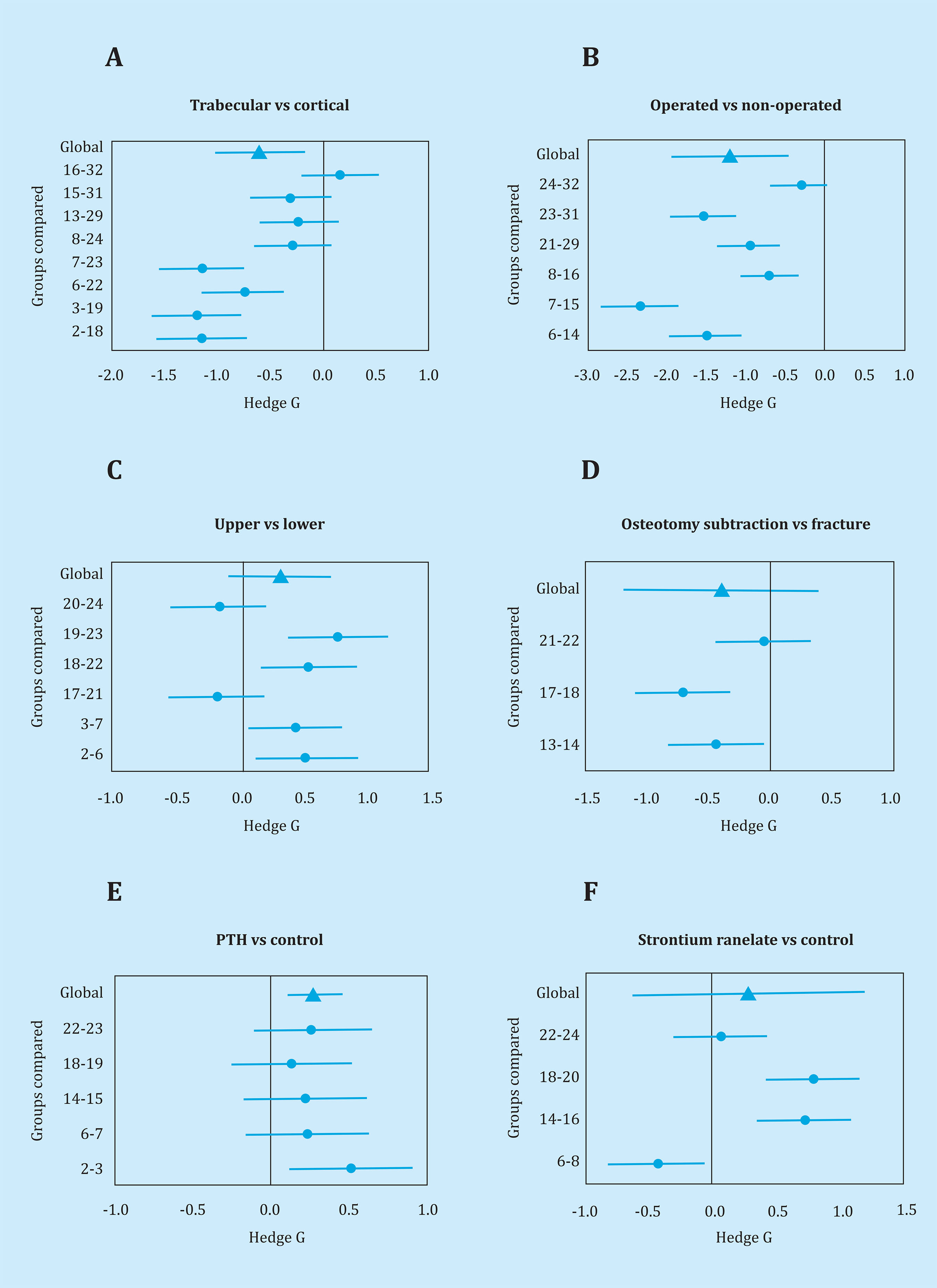
Figure 1. Summary of the results obtained in the UMI tests. Hedge g values of the different comparisons between groups. The average value (similar to the standardized mean difference) and the 95% confidence interval of each comparison (circles) are shown. The global value and its confidence interval (triangles) are also included. The numbers on the left axis identify the groups compared, as designated in table 1
As for regional variability, the hardness of the material was consistently greater in cortical bone than in trabecular bone, with an average difference of approximately 0.6 standard deviations (p=8.0x10-4, figure 1A). Similarly, the hardness was consistently greater in the upper femoral epiphyses than in the lower epiphyses (standard difference 1.2 units, p=5x10-5, figure 1C).
The possible impact of the surgical intervention at the regional level was explored by comparing the hardness in the operated and non-operated femurs. As shown in figure 1B, there was a non-significant trend for the decrease in hardness in the operated femur (difference of 0.3 standard deviations, p=5.5x10-2). The standard fracture could only be compared with SO in three groups. This last procedure tended to be associated with a lower hardness (p=3.7x10-2, figure 1D).
The effect of PTH was explored in five pairs of groups. The drug induced a slight but consistent increase in hardness at all sites (p=1.8x10-5, figure 1E). However, the effect of SR was inconsistent (p=3.0x-10-1, figure 1F).
DISCUSSION
From a clinical perspective, bone mass evaluated by DXA is the most widely used predictor of bone's ability to withstand the repetitive burdens of daily life and other occasional impacts. However, from a mechanical point of view, bone architecture (the distribution of bone mass) and quality (that is, the intrinsic material properties of tissue), are the relevant determinants of global bone strength.
Advanced imaging techniques, such as high resolution computerized tomography (CT) and nuclear magnetic resonance imaging (MRI), can provide useful information about bone geometry, cortical porosity and trabecular microarchitecture. However, bone quality remains a somewhat elusive concept, because biochemical and cellular determinants are incompletely known and not easy to measure. Bone hardness (expressing resistance to plastic deformation) is often used as a marker of tissue quality. In this sense, the determination of hardness is emerging as a technique that provides useful information in clinical studies11. UMI tests allow us to obtain other parameters of interest, in addition to hardness, to characterize the mechanical behavior of bone tissue, in particular Young's elastic modulus of the material. However, the available evidence shows that the definition of the test parameters can play a relevant role in the results obtained. In the work of Zhang et al.12the values obtained for hardness and Young's modulus in bones are compared from nanoindentation and microindentation tests. According to these authors, while hardness is a stable parameter against load values, Young's module is significantly reduced by increasing the load value. For this reason, hardness is preferable when carrying out comparisons with other studies.
Due to practical and ethical issues, hardness is usually determined at a single bone point. However, there is little information about the differences in hardness evaluated in different skeletal regions and about the influence of diseases and pharmacological therapies. Therefore, we take advantage of a rat fracture study to try to provide additional information in this important field of research. Our data clearly shows that the hardness is consistently greater in cortical bone than in trabecular bone. Similarly, it is different through individual bones, and specifically, it is greater in the superior femoral epiphyses than in the inferior ones. On the other hand, induced fracture tends to decrease hardness in fractured bone, while PTH increases hardness in all regions analyzed.
Since the determinants of bone hardness have not been fully clarified, the mechanisms underlying these differences remain unknown. However, it is not risky to speculate that they must be related to the matrix composition and, specifically, to its main organic and inorganic components, namely collagen and hydroxyapatite. In fact, the suggested determinants of the mechanical behavior of bone tissue include: collagen orientation, collagen cross-linking profile, degree of mineralization or mineral-matrix ratio, bound water and mineral structure (including the size of the hydroxyapatite crystals)13. The orientation of the collagen fiber may be one of the factors responsible for the differences observed between the hardness of the trabecular bone and the cortical bone. Also, different remodeling rates can play a role.
Thus, the increase in tissue age is associated with greater microhardness, perhaps due to greater mineralization14. Similarly, changes in bone remodeling induced by an injury can help explain the differences we find between the operated and the non-operated femur. The mechanical load has a known anabolic effect on the bone. Therefore, increased load is usually associated with increased bone mass, while discharge causes a rapid loss of bone density. It is less known that mechanical stimulation can cause changes in bone quality7. Although the real relevance of such an effect is still unclear, it can also help explain the differences we have observed between skeletal regions and between groups undergoing various interventions. In particular, the lower support of the intervened limb, and consequently the submission to a lower mechanical load, can help explain the lower hardness observed in fractured femurs.
Intermittent administration of PTH or related molecules that activate the PTH receptor, such as teriparatide or abaloparatide, decreases the risk of fracture. The effect of PTH on tissue hardness is controversial. Brennan et al.15and Amugongo et al.16reported absence of changes in microhardness in ovariectomized rats treated with teriparatide. On the other hand, Mellibovsky et al. indicated that teriparatide improved the properties of the material in patients with glucocorticoid-induced osteoporosis11. In this study we found a small but significant effect of PTH on tissue hardness, probably related to PTH-induced changes in bone remodeling17,18.
It is interesting to note that, although proteins other than collagen represent only a small fraction of the bone matrix, around 10%, they seem important in determining bone hardness and strength. On the one hand, they contribute to regulate mineralization. On the other, they create bonds with collagen fibers that help absorb and dissipate energy at the nano-structural level19. Certainly, some treatments may induce changes in the expression of the genes encoding these proteins, as well as in the amount and spatial distribution of hydroxyapatite crystals, thus constituting another mechanism by which to influence the mechanical properties of the bone matrix.
In summary, although tissue composition and microstructure are probably important factors of tissue resistance, material properties also contribute to bone strength. Our study shows that tissue microhardness is influenced by a variety of factors, including anatomy, type of bone tissue, skeletal injury and drug therapy. Therefore, future studies on tissue quality should be carefully designed with these factors in mind.
REFERENCES
1 Rachner TD, Khosla S, Hofbauer LC. Osteoporosis: now and the future. Lancet. 2011;377:1276-87. [ Links ]
2 Ritchie RO. How does human bone resist fracture? Ann N Y Acad Sci. 2010; 1192:72-80. [ Links ]
3 Ammann P, Rizzoli R. Bone strength and its determinants. Osteoporos Int. 2003;14 (suppl):S13-8. [ Links ]
4 Fonseca H, Moreira-Gonçalves D, Coriolano H, Duarte J. Bone quality: the determinants of bone strength and fragility. Sport Med. 2014;44:37-53. [ Links ]
5 Rho JY, Kuhn-Spearing L, Zioupos R. Mechanical properties and the hierarchical structure of bone. Nature. 1998; 20:92-102. [ Links ]
6 Gupta HS, Zioupos P. Fracture of bone tissue: The "hows" and the "whys". Med Eng Phys. 2008;30:1209-26. [ Links ]
7 Berman AG, Wallace JM. Bone quality and quantity are mediated by mechanical stimuli. Clin Rev Bone Miner Metab. 2016;14:218-26. [ Links ]
8 Hunt HB, Donnelly E. Bone quality assessment techniques: geometric, compositional, and mechanical characterization from macroscale to nanoscale. Clin Rev Bone Miner Metab. 2016;14:133-49. [ Links ]
9 Guerri-Fernández RC, Nogues X, Quesada Gómez JM, Torres DP, Puig L, García-Giralt N, et al. Microindentation for in vivo measurement of bone tissue material properties in atypical femoral fracture patients and controls. J Bone Miner Res. 2013;28:162-8. [ Links ]
10 Pérez Núñez MI, Ferreño Blanco D, Alfonso Fernández A, Casado del Prado JA, Sánchez Crespo M, de la Red Gallego M, et al. Comparative study of the effect of PTH (1-84) and strontium ranelate in an experimental model of atrophic nonunion. Injury. 2015;46: 2359-67. [ Links ]
11 Mellibovsky L, Prieto-Alhambra D, Mellibovsky F, Guerri-Fernández R, Nogues X, Randall C, et al. Bone tissue properties measurement by reference point indentation in glucocorticoid-induced osteoporosis. J Bone Miner Res. 2015;30:1651-6. [ Links ]
12 Zhang J, Niebur GL, Ovaert TC. Mechanical property determination of bone through nano- and micro-indentation testing and finite element simulation. J Biomech. 2008;41:267-75. [ Links ]
13 Unal M, Creecy A, Nyman JS. The Role of matrix composition in the mechanical behavior of bone. Curr Osteoporos Rep. 2018;16:205-15. [ Links ]
14 Donnelly E, Boskey A, Baker S, van der Meulen M. Effects of tissue age on bone tissue material composition and nanomechanical properties in the rat cortex. J Biomed Mater Res A. 2010; 92:1048-56. [ Links ]
15 Brennan TC, Rizzoli R, Ammann P. Selective modification of bone quality by PTH, pamidronate, or raloxifene, J Bone Miner Res. 2009;24:800-8. [ Links ]
16 S. Amugongo S, Yao W, Jia J, Dai W, Lay Y, Jiang L, et al. Effect of sequential treatments with alendronate, parathyroid hormone (1-34) and raloxifene on cortical bone mass and strength in ovariec-tomized rat. Bone. 2014;67:257-68. [ Links ]
17 Burr DB. Bone biomechanics and bone quality: effects of pharmaceutical agents used to treat osteoporosis. Clin Rev Bone Miner Metab. 2016;14:197-217. [ Links ]
18 Imbert I, Boskey A. Effects of drugs on bone quality. Clin Rev Bone Miner Metab. 2016;14:167-96. [ Links ]
19 Gardinier J, Al-Omaishi S, Rostami N, Morris M, Kohn D. Examining the influence of PTH(l-34) on tissue strength and composition. Bone. 2018;117:130-7. [ Links ]
Acknowledgments
This study was partially supported by research grants from the Marqués de Valdecilla Research Institute, Nycomed and Servier.
Ethical statement: This work was carried out in accordance with the guidelines of the University of Cantabria and in accordance with EU Directive 2010/63/EU http://eur-lex.europa.eu/legal-content/EN/TXT/?Uri=CELEX:32010L0063.
Received: December 07, 2019; Accepted: January 22, 2020