My SciELO
Services on Demand
Journal
Article
Indicators
-
Cited by SciELO
-
Access statistics
Related links
-
Cited by Google
-
Similars in SciELO
-
Similars in Google
Share
Revista de Osteoporosis y Metabolismo Mineral
On-line version ISSN 2173-2345Print version ISSN 1889-836X
Rev Osteoporos Metab Miner vol.12 n.1 Madrid Jan./Mar. 2020 Epub June 15, 2020
https://dx.doi.org/10.4321/s1889-836x2020000100005
ORIGINAL ARTICLES
Influence of high-concentration hyperbaric oxygen therapy on bone metabolism
1Internal Medicine Service. Marqués de Valdecilla University Hospital. Valdecilla Research Institute (IDIVAL). University of Cantabria. Santander (Spain).
2Radiation Oncology Service. Marqués de Valdecilla University Hospital. Valdecilla Research Institute (IDIVAL). University of Cantabria. Santander (Spain).
3Intensive Medicine Service. Marqués de Valdecilla University Hospital. Valdecilla Research Institute (IDIVAL). University of Cantabria. Santander (Spain).
4Traumatology and Orthopedics Service. Marqués de Valdecilla University Hospital. Valdecilla Research Institute (IDIVAL). University of Cantabria. Santander (Spain).
Objectives:
To learn how high concentration in hyperbaric oxygen therapy (HBO) acts on the expression of genes related to bone metabolism in osteoblast cell lines and human trabecular bone.
Material and methods:
The differential expression of several genes related to bone metabolism (SOST, RUNX2, MMP14, OPG, HIF-1α and SIRT1) in two human osteoblastic cell lines (Saos and Super-Saos) and in human trabecular bone fragments subjected to one, three or five HBO sessions (90 minutes, 100% oxygen; 2.3 atmospheres). In each experiment, a control that did not receive HBO was used.
Results:
We did not find significant differences after HBO in the expression of the genes studied, neither in the cells nor in trabecular bone. Only in the Super-Saos cell line the expression of OPG after 5 sessions of HBO decreased 6 times with respect to that of the control group (2-ΔCt Ct of 72; p=0.01).
Conclusions:
High concentration oxygen in the hyperbaric chamber (HC) does not seem to influence the expression of
genes related to bone metabolism.
Key words: Oxygen; hyperbaric chamber; bone; genes
INTRODUCTION
Oxygen is required to produce cellular energy and is involved in numerous processes, such as enzymatic activation, molecular signaling and regulation of gene expression1. Also in angiogenesis, the maintenance of hematopoietic stem cells and bone formation2. In fact, changes in the partial pressure of oxygen can influence the function of osteoblasts and osteoclasts3. In hypoxia, bone formation and mineralization decreases, while resorption increases4-6. In the opposite direction, hyperoxia could have a beneficial effect on the bone. Treatment with high concentration of oxygen in the hyperbaric chamber has proven useful in osteomyelitis and osteonecrosis of the jaw caused by radiotherapy or by the use of bisphosphonates7-9. HC accelerates osteogenic differentiation of mesenchymal cells and decreases the activation of osteoclasts10-12.
In this work we wanted to analyze the actions of oxygen at high concentration in HBO on the expression of genes related to bone metabolism in osteoblastic cell lines and human bone5,6,13,14.
Material and methods:
Cell lines
Two osteoblastic cell lines, Saos-2 and Super-Saos, were used. Saos-2, derived from a human osteosarcoma. Super-Saos is a line generated in our laboratory, derived from the previous one and with a high capacity to express the sclerostin gene (SOST)15. Both lines were grown in T25 bottles with 5 ml of DMEM culture medium (Dulbeco's modified Eagle culture medium) plus 1% P/S (penicillinstreptomycin) and 1% amphotericin B, and stored in an incubator at 37°C for one week, changing the culture medium every 4 days to cover between 60-80% of the surface of the bottle. The plates were introduced into the HC (Galeazzi, Italy; 100% oxygen; 2.4 atmospheres) for 90 minutes per session receiving one, three or five consecutive sessions (Figure 1). The same cell line was used as control group subjected to identical culture, transport and handling conditions, but without undergoing HBO.
Bone fragments
Trabecular bone fragments extracted from the femoral head of patients with osteoporotic fracture hip replacement surgery were used. After extraction, the bone fragments received a single session of HC (Galeazzi, Italy; 100% oxygen; 2.4 atmospheres) for 90 minutes and subsequently frozen at -70°C. Bone fragments subjected to the same conditions of conservation, cul ture, transport and handling were used as controls but without receiving HC. This experiment was approved by the Clinical Research Ethics Committee (CEIC) of Cantabria. All patients gave informed consent.
RNA extraction and quantification
24 hours after the last HBO session, RNA was extracted, both in the cell lines and in the bone. In the homogenization process in cell lines, the samples were washed with phosphate buffered saline (PBS) prior to the use of TRIzol®. In the case of bone fragments, TRIzol® was also used, as well as homogenization for 20-30 seconds until the sample was pulverized, and subsequently centrifuged. In both cases the manufacturer's recommendations were followed and the RNA separation, precipitation and resuspension process continued.
Quantitative RT-PCR (polymerase chain reaction with reverse transcriptase) was carried out to detect gene expression: SOST (sclerostin gene), RUNX2 (protein related to transcription factor 2), MMP14 (metalloproteinase 14), HIF-1α (hypoxia-inducible factor), SIRT1 (sirtuin1), OPG (osteoprotegerin) and RANKL (kappa-nuclear nuclear factor receptor activator ligand) using Taqman assays and following the manufacturer's instructions. The threshold cycle (Ct) values were obtained and the data normalized to the expression of GAPDH (glyceraldehyde-3-phosphate dehydrogenase) and TBP (TATA box binding protein) using the ΔCt method. To calculate the relative level of mRNA, the formula 2-ΔCt was used, where ΔCt is the difference between the Ct average of the normalizing genes and the Ct of the gene of interest.
Results:
HBO effect on RNA expression in the Saos-2 cell line
There were no differences in the expression of genes in the cell line after one, three or five HBO sessions. The differences with respect to the control in 2-ΔCt after 5 sessions were 0.71 for SOST (p=0.50), 0.89 for SIRT1 (p=0.34), 0.47 for MMP14 (p=0.18), 0.43 for HIF1α (p=0.18), 0.79 for RUNX2 (p=0.65) and 7.91 for OPG (p=0.40) (Figure 2). No RANKL expression was detected.
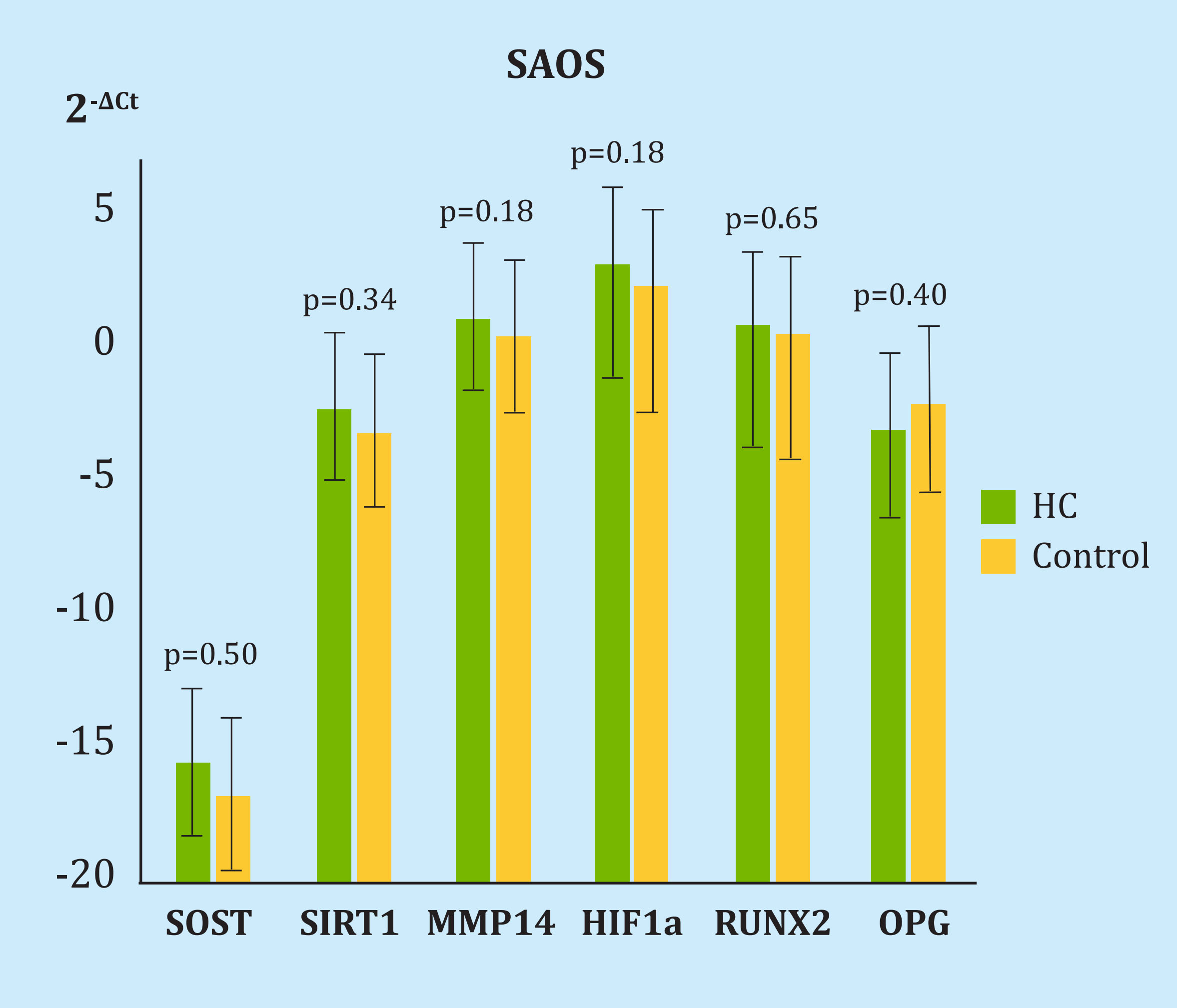
HC: cell group undergoing hyperbaric oxygen therapy; SOST: sclerostin; SIRT1: sirtuin1; MMP14: metalloproteinase 14; HIF-1α: hypoxia inducible factor 1α; RUNX2: protein related to transcription factor 2; OPG: osteoprotegerin.
Figure 2. Difference in the expression of genes under study and housekeeping in the SAOS cell line after 5 sessions of hyperbaric chamber
Effect of HBO on RNA expression in the Super-SaOS cell line
Compared to the control, we found OPG expression decreases 6 times after 5 HBO sessions (2-ΔCt, 72 p=0.01). In the rest of the genes there were no differences: 2-ΔCt, from 1.03 for SOST (p=0.34), 1.46 for SIRT1 (p=0.34), 1.77 for MMP14 (p=0.18), 1.08 for HIF1α (p=0.18), 1.14 for RUNX2 (p=0.18) and 1.24 for RANKL (p=0.31) (Figure 3).
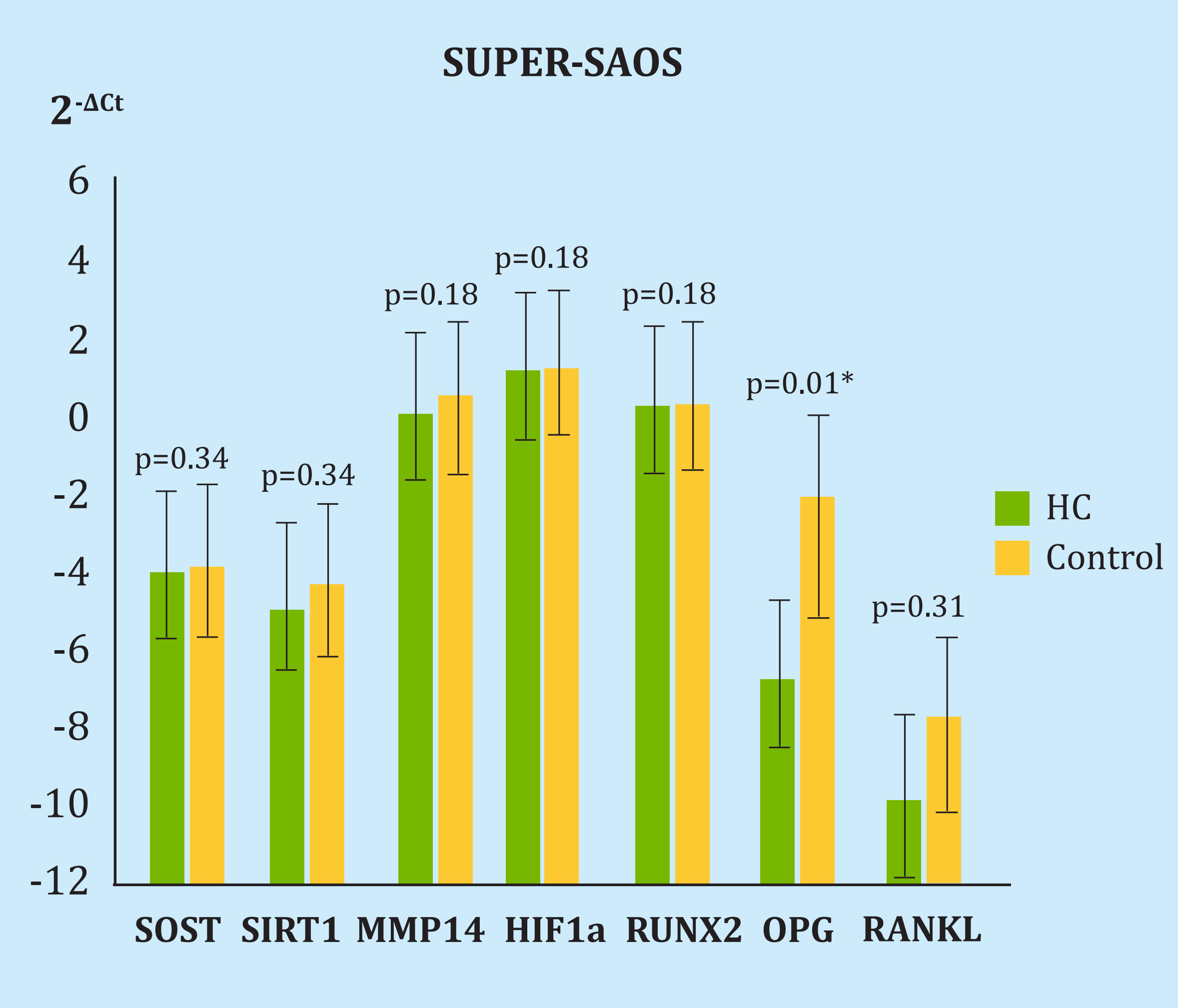
HC: cell group undergoing hyperbaric oxygen therapy; SOST: sclerostin; SIRT1: sirtuin1; MMP14: metalloproteinase 14; HIF-1α: hypoxia inducible factor 1α; RUNX2: protein related to transcription factor 2; OPG: osteoprotegerin.
Figure 3. Difference in the expression of genes under study and housekeeping in the Super-SAOS cell line after 5 sessions of hyperbaric chamber
HBO effect on RNA expression in trabecular bone
Nor were there differences in the expression of genes after HBO in bone, only a modest, non-significant increase in the expression of SOST with a 2-ΔCt change of 5.39 (p=0.48). In the rest of the genes the differences were 0.92 for MMP14 (p=0.58), 1.28 for HIF1α (p=0.81), 0.72 for RUNX2 (p=0.24), 1, 18 for SIRT1 (p=0.42), 1.97 for RANKL (p=0.91) and 3.9 for OPG (p=0.55) (Figure 4).
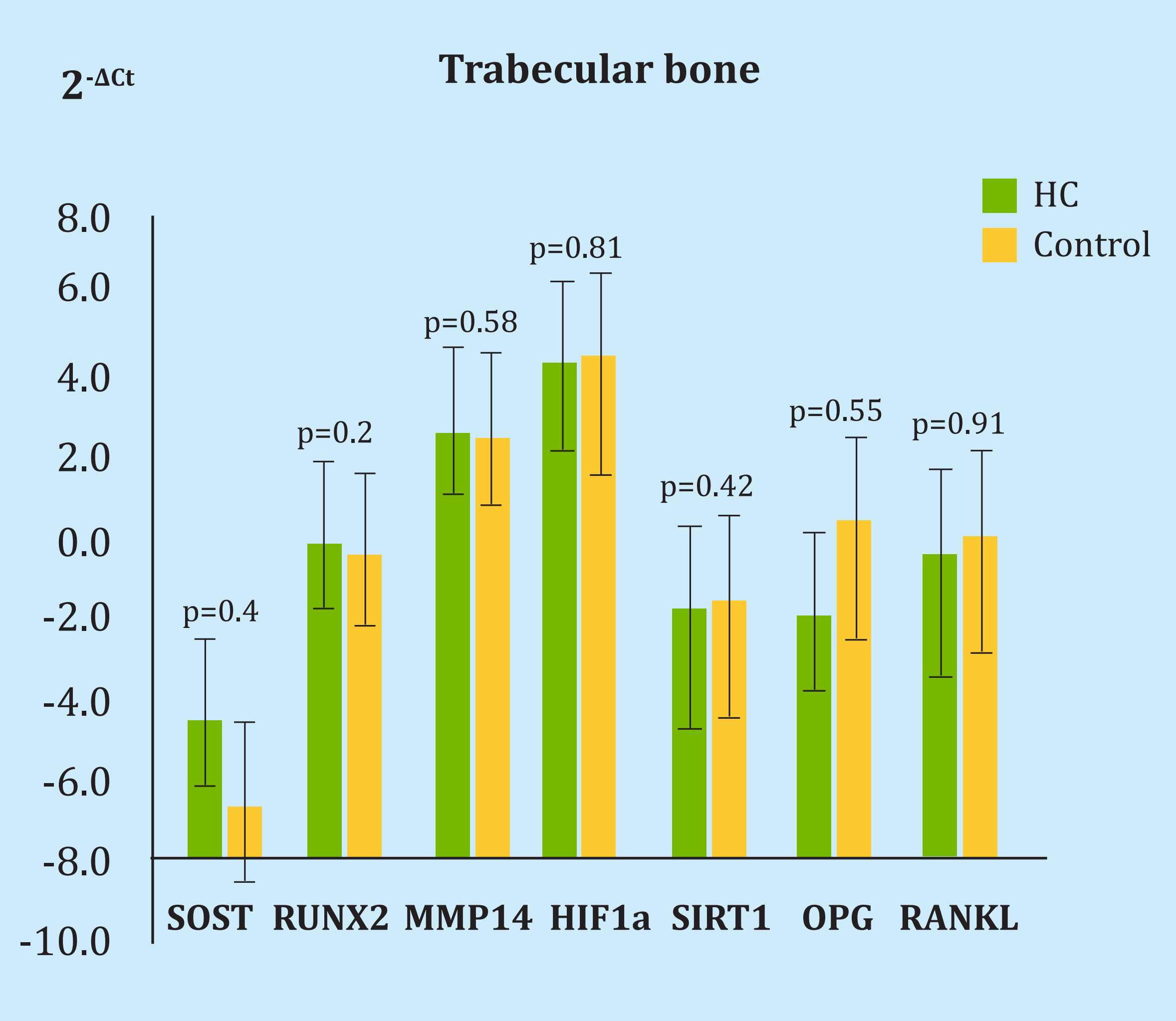
HC: cell group undergoing hyperbaric oxygen therapy; SOST: sclerostin; SIRT1: sirtuin1; MMP14: metalloproteinase 14; HIF-1α: hypoxia inducible factor 1α; RUNX2: protein related to transcription factor 2; OPG: osteoprotegerin.
Figure 4. Difference in the expression of genes under study and housekeeping in bone after a single hyperbaric oxygen therapy session
Conclusions:
Hyperoxia is considered beneficial for bone by increasing the proliferation and differentiation of osteoblasts16. Al Hadi et al.6 described increased expression of type I collagen and Runx-2 mRNA in osteoblast cell lines (Saos-2) subjected to HBO for 14 days (2.4 ATA, 97% O2, 90 min/day). HBO also increased the proliferation and differentiation of osteoblasts in human alveolar bone17. Hyperoxia also seems to decrease bone resorption. Treatment in HC (100% O2, 2.4 ATA) reduced the expression of RANK, NFATc1 and Dc-STAMP in the serum of patients and also regulated the expression of the hypoxia inducible factor (HIF-1α)18. Other described actions of oxygen at high concentration (100% O2, 2.4 ATA) are the improvement in angiogenesis, increased vascularization of the aspirated iliac crest of mice19, greater cell proliferation20 or acceleration in the healing of open femoral fractures in experimental animals21. However, most of these works have been carried out in animal models and human studies are scarce. In patients with avascular necrosis of the femoral head, serum OPG levels increased after HBO (5.61±1.99 pmol/L at baseline, 7.90±1.9 pmol/L after 15 sessions, 8.97±2.07 pmol/L after 30 sessions; p<0.05), without changes in RANKL22 levels. After HBO (2.5 ATA, 100% O2 for 90 min/day), osteogenic differentiation of bone marrow mesenchymal cells was also improved in treated patients, with an up-regulation in Wnt3a, b-catenin and Runx2 and descending GSK-3b, compared to those who did not receive it12. These same authors also described an increase in bone morphogenetic protein (BMP2) and Osterix in treated patients12.
In our study, we did not find that oxygen at high concentration in HC influences the expression of different genes related to bone metabolism (SOST, SIRT1, MMP14, HIF1a, RUNX2, OPG and RNAKL). However, we would highlight that we found a slight tendency, not significant, to the increase in the expression of SOST in the bone undergoing treatment. We know that oxygen tension influences the regulation of SOST and that in hypoxia (1% oxygen tension) osteoblasts and osteocytes express low levels of SOST and sclerostin23, perhaps this is due to a lower expression of prolyl hydroxylase (PHD2) since it has been seen that deletion of PHD2 in osteocytes causes a lower production of sclerostin dependent on SIRT114. This pathway could elucidate our understanding of the pathophysiological mechanism through which, and in the opposite direction, an oxygen-rich environment could increase the expression of SOST and sclerostin. In fact, our group has found a 25% increase in serum sclerostin levels in 12 patients undergoing HBO treatment. However, other works are contradictory13.
In conclusion, it does not appear that hyperoxia in HC influences the expression of genes related to bone metabolism, although we believe that more studies are needed to broaden our knowledge of the actions of oxygen in bone.
REFERENCES
1. Johnson RW, Sowder ME, Giaccia AJ. Hypoxia and bone metastatic disease. Curr Osteoporos Rep [Internet]. 2017;15(4):231-8. [ Links ]
2. Parmar K, Mauch P, Vergilio J-A, Sackstein R, Down JD. Distribution of hematopoietic stem cells in the bone marrow according to regional hypoxia. Proc Natl Acad Sci. 2007;104(13):5431-6. [ Links ]
3. Kusumbe AP, Ramasamy SK, Adams RH. Coupling of angiogenesis and osteogenesis by a specific vessel subtype in bone. Nature. 2014;507 (7492):323-8. [ Links ]
4. Arnett TR. Acidosis, hypoxia and bone. Arch Biochem Biophys. 2010;503(1): 103-9. [ Links ]
5. Utting JC, Flanagan AM, Brandao-Burch A, Orriss IR, Arnett TR. Hypoxia stimulates osteoclast formation from human peripheral blood. Cell Biochem Funct. 2010;28(5):374-80. [ Links ]
6. Al Hadi H, Smerdon GR, Fox SW. Hyperbaric oxygen therapy accelerates osteoblast differentiation and promotes bone formation. J Dent. 2015; 43(3):382-8. [ Links ]
7. Ceponis P, Keilman C, Guerry C, Freiberger JJ. Hyperbaric oxygen therapy and osteonecrosis. Oral Diseases. 2017;23(2):141-51. [ Links ]
8. Beth-Tasdogan NH, Mayer B, Hussein H, Zolk O. Interventions for managing medication-related osteonecrosis of the jaw. Cochrane Database Syst Rev. 2017;10:CD012432. [ Links ]
9. Eltorai I, Hart GB, Strauss MB. Osteomyelitis in the spinal cord injured: A review and a preliminary report on the use of hyperbaric oxygen therapy. Paraplegia. 1984;22(1):17-24. [ Links ]
10. Al Hadi H, Smerdon GR, Fox SW. Hyperbaric oxygen therapy suppresses osteoclast formation and bone resorption. J Orthop Res. 2013;31[11):1839-44. [ Links ]
11. Lin S-S, Ueng SW, Niu C-C, Yuan L-J, Yang C-Y, Chen W-J, et al. Effects of hyperbaric oxygen on the osteogenic differentiation of mesenchymal stem cells. BMC Musculoskelet Disord. 2014;15(1):56. [ Links ]
12. Lin S-S, Ueng SWN, Niu C-C, Yuan L-J, Yang C-Y, Chen W-J, et al. Hyperbaric oxygen promotes osteogenic differentiation of bone marrow stromal cells by regulating Wnt3a/β-catenin signa-ling--an in vitro and in vivo study. Stem Cell Res. 2014;12[1):260-74. [ Links ]
13. Fujiwara M, Kubota T, Wang W, Ohata Y, Miura K, Kitaoka T, et al. Successful induction of sclerostin in human-derived fibroblasts by 4 transcription factors and its regulation by parathyroid hormone, hypoxia, and prostaglandin E2. Bone. 2016;85:91-8. [ Links ]
14. Stegen S, Stockmans I, Moermans K, Thienpont B, Maxwell PH, Carmeliet P, et al. Osteocytic oxygen sensing controls bone mass through epigenetic regulation of sclerostin. Nat Commun. 2018;9(1):2557. [ Links ]
15. Pérez-Campo FM, Sañudo C, Delgado-Calle J, Arozamena J, Zarrabeitia MT, Riancho JA. A sclerostin super-producer cell line derived from the human cell line SaOS-2: a new tool for the study of the molecular mechanisms driving sclerostin expression. Calcif Tissue Int. 2014;95(2):194-9. [ Links ]
16. Chang H, Oh S-E, Oh S, Hu K-S, Kim S. Four-week histologic evaluation of grafted calvarial defects with adjunctive hyperbaric oxygen therapy in rats. J Periodontal Implant Sci. 2016; 46(4):244-53. [ Links ]
17. Wu D, Malda J, Crawford R, Xiao Y Effects of hyperbaric oxygen on proliferation and differentiation of osteoblasts from human alveolar bone. Connect Tissue Res. 2007;48(4):206-13. [ Links ]
18. Al Hadi H, Smerdon G, Fox SW. Osteoclastic resorptive capacity is suppressed in patients receiving hyperbaric oxygen therapy. Acta Orthop. 2015;86(2):264-9. [ Links ]
19. Grassmann JP, Schneppendahl J, Sager M, Hakimi AR, Herten M, Loegters TT, et al. The effect of bone marrow concentrate and hyperbaric oxygen therapy on bone repair. J Mater Sci Mater Med. 2015;26(1):5331. [ Links ]
20. Sunkari VG, Lind F, Botusan IR, Kashif A, Liu Z-J, Ylä-Herttuala S, et al. Hyperbaric oxygen therapy activates hypoxia-inducible factor 1 (HIF-1), which contributes to improved wound healing in diabetic mice. Wound Repair Regen. 2015;23(1):98-103. [ Links ]
21. Kawada S, Wada E, Matsuda R, Ishii N. Hyperbaric hyperoxia accelerates fracture healing in mice. PLoS One. 2013;8(8):e72603. [ Links ]
22. Vezzani G, Quartesan S, Cancellara P, Camporesi E, Mangar D, Bernasek T, et al. Hyperbaric oxygen therapy modulates serum OPG/RANKL in femoral head necrosis patients. J Enzyme Inhib Med Chem. 2017;32[1):707-11. [ Links ]
23. Genetos DC, Toupadakis CA, Raheja LF, Wong A, Papanicolaou SE, Fyhrie DP, et al. Hypoxia decreases sclerostin expression and increases Wnt signaling in osteoblasts. J Cell Biochem. 2010; 110(2):457-67. [ Links ]
Received: January 08, 2020; Accepted: February 24, 2020