Meu SciELO
Serviços Personalizados
Journal
Artigo
Indicadores
-
Citado por SciELO
-
Acessos
Links relacionados
-
Citado por Google
-
Similares em SciELO
-
Similares em Google
Compartilhar
Revista de Osteoporosis y Metabolismo Mineral
versão On-line ISSN 2173-2345versão impressa ISSN 1889-836X
Rev Osteoporos Metab Miner vol.15 no.3 Madrid Jul./Set. 2023 Epub 08-Mar-2024
https://dx.doi.org/10.20960/revosteoporosmetabminer.00013
REVIEW
Cellular senescence as a pathogenic factor and potential therapeutic target in osteoporosis
1Department of Traumatology and Orthopedic Surgery. Hospital Comarcal García Orcoyen. Estella, Navarra, Spain
2Clinical Management Unit of Bone Metabolism. Hospital Universitario Central de Asturias. Oviedo, Spain
3Clinical Management Unit of Bone Metabolism. Hospital Universitario Central de Asturias
3Grupo de Metabolismo Óseo, Vascular y Enfermedades Inflamatorias Crónicas. Instituto de Investigación Sanitaria del Principado de Asturias (ISPA)
3RICORS 2040 - Enfermedad Renal del ISCIII. Oviedo
Cellular senescence is a process induced by various types of stress that irreversibly cause cell cycle arrest and changes to the characteristics and functionality of cells, as well as the acquisition of a secretory phenotype that generates a pro-inflammatory environment. While, in certain contexts, it is beneficial for tissues and promotes organism development, senescence is a cellular fate implicated in the process of aging and age-related degenerative conditions. Senolytics are drugs that specifically eliminate senescent cells, and senomorphics are drugs that suppress their senescence-associated secretory phenotype (SASP) without inducing cell death. Therefore, therapeutic strategies targeting senescent cells (senolytics and senomorphics) as an underlying mechanism of aging emerge as an alternative with great potential to fight age-related diseases as a whole rather than individually. One of these conditions is osteoporosis where it has been experimentally described that drugs such as zoledronic acid have effects on preosteoblasts and act on senescent cells extending survival and opening up the possibility of treating age-related diseases with drugs already used in practice, which may have effects beyond the bone itself and increase overall survival. In this study, a review will be conducted in this rapidly growing field in recent years of undeniable translational interest.
Keywords: Senescence; Osteoporosis; Senolytics; Senomorphics; Bone; Frailty; Senescence-associated secretory phenotype (SASP)
INTRODUCTION
The remarkable increase in life expectancy since the mid-20th century has led to a rapid aging of the population (1). Globally, the population over 65 years old is growing at a faster pace compared to other population segments. As a matter of fact, it is estimated that by 2050, the overall number of octogenarians will triple the numbers from 2019 (2).
This increase in longevity, an achievement of improved life conditions and medicine itself, is accompanied by an increase in the burden of chronic diseases (cardiovascular, musculoskeletal, neoplastic, and neurological) (3-5), which not only has social and economic implications but also results in a loss of quality of life, functional limitations, frailty, and mortality (6,7). In the current context, developing strategies with a focus on treating aging as the common denominator of these conditions, rather than each specific disease, can be of tremendous relevance, extending health and delaying, preventing, or alleviating age-related disorders.
The cellular mechanisms involved in aging include telomere shortening, genomic instability, epigenetic alterations, mitochondrial dysfunction, loss of protein homeostasis (proteostasis), depletion and decline of stem cells, nutrient sensing deregulation, immune system decline, and cellular senescence (8,9). These processes are interrelated (10), linked and overlap. Senescence is not only a cause but also a consequence of several of them with senescent cells acting as true “synchronizers” of aging in different tissues, organs, or systems. Therefore, the objective of this work was to conduct a review in this rapidly growing field in recent years of undeniable translational interest.
CONCEPT
Cellular senescence is defined as a stable state of cell cycle arrest in response to different stimuli, in which cells cease proliferation and acquire an altered phenotype, thus losing their primary functionality. The concept was first described back in 1961 by Hayflick et al. (11) in human diploid fibroblasts cultured in vitro. It was demonstrated that normal cells have a limited capacity for division due to telomere shortening. This phenomenon is now known as replicative senescence, although many other stress stimuli triggering cellular senescence have been discovered.
Overall, types of senescence can be grouped based on their triggers into replicative senescence, DNA damage-induced senescence, oncogene-induced senescence, oxidative stress-induced senescence, mitochondrial dysfunction-associated senescence, epigenetically induced senescence, paracrine senescence, and endocrine senescence (induced by the inflammatory environment generated by senescent cells themselves) (12). DNA damage such as telomere shortening and single- and double-strand breaks, oncogenic mutations (eg, Ras, Myc, B-Raf) that typically affect genes involved in cell cycle control, reactive metabolites like reactive oxygen species (ROS) and bioactive lipids, signals of elevated mitogens and nutrients that increase the mammalian target of rapamycin (mTOR) activity, proteotoxic stress such as protein aggregation and unfolded proteins, and damage-associated molecular patterns (DAMPs), among others, have been found to induce senescence. Most of these findings have been demonstrated in cell culture experiments and have been considered inducers of senescence in vivo ever since (13).
All these effectors contribute to widespread changes in gene expression, metabolism, and chromatin organization behind the growth arrest associated with senescence, structural changes in cells, and a specialized secretory activity known as the senescence-associated secretory phenotype (SASP). Additionally, it generates an inflammatory environment. Structural changes described in senescent cells include flattened, vacuolated, and enlarged morphology, altered composition of the plasma membrane, and accumulation of lysosomes and mitochondria (14).
PHYSIOLOGY AND PATHOPHYSIOLOGY OF SENESCENCE
The general objective of senescence is the elimination of unwanted cells in the body. Therefore, its involvement in cancer defense is vital: in response to oncogenic agents, senescence is induced, and replication of cancer cells is halted (the proapoptotic SASP can even eliminate surrounding tumor cells) (3,10). Under physiological conditions, it plays a key role in the response to damage or stress and in tissue repair and remodeling, such as in wound healing processes (15). Its physiological role in embryonic development is well-established, as it participates in the formation of various anatomical structures during organogenesis and regulates the proportion of different cell types (complementing apoptosis). It also occurs in healthy adult tissues as a mechanism for the maturation of megakaryocytes and syncytiotrophoblasts in the placenta and for organism protection (12,13).
When senescent cells are not efficiently eliminated and accumulate, far from promoting regeneration, they exacerbate tissue dysfunction and contribute to the genesis of diseases (16,17). Senescence not only occurs with age but also drives aging by synchronizing it in different tissues and systems (18,19).
From the above, it can be deduced that cellular senescence is a complex phenomenon that can act as a defense mechanism to halt disease progression (3) under physiological conditions, while in other situations, it may promote disease development. When localized and limited in time, it promotes tissue remodeling during growth or after tissue damage, but it also contributes to the decline of the regenerative and functional potential of tissues, inflammation, and tumorigenesis when pronounced or persistent, as seen in aged organisms.
SIGNALING PATHWAYS OF SENESCENCE
From a molecular perspective, cellular senescence can be understood as a cellular fate that occurs at any point in life and involves the action of external and internal inducers, transcription factor cascades, changes in gene expression, and chromatin remodeling (20). Initially, the activation of the p53/p21Cip1 and tumor suppressor p16INK4a pathways occurs, generating a response that takes time to fully establish and is irreversible. The process is reinforced by an intracellular signaling loop that includes ROS (reactive oxygen species) linked to DNA damage responses, NFκB (nuclear factor kappa B), TGF-β (transforming growth factor beta), and GATA4 (guanine adenine thymine adenine), as well as an IL-1α, IL-6, and CCAAT-enhancer-binding protein beta (C/EBP-β) loop (5).
The senescent phenotype acquired by these cells is generally accompanied by an increased secretion of proinflammatory factors: TGF-β, NFκB, IL-1α, IL-6, IL-8, chemokines that draw and anchor immune cells, and activation of different enzymes such as metalloproteinases. Other characteristics of the senescent phenotype include nuclear expression of cell cycle inhibitors and tumor suppressors (p15, p16, p21, p27, p53, hypo-phosphorylated Rb), absence of proliferative markers like Ki67, expression of DNA damage markers, presence of senescence-associated heterochromatin foci (SAHF) in the nucleus, and accumulation of lipofuscin (20). As mentioned, senescent cells upregulate several antiapoptotic pathways known as senescent cell anti-apoptotic pathways (SCAPs): BCL-2/BCL-w/BCL-XL family pathway, PI3K (phosphatidylinositol-3-kinase)/Akt pathway, p53/p21/serpine pathway, ephrins/dependence receptor/tyrosine kinase pathway, HIF-1α (hypoxia-inducible factor 1 alpha) pathway, and HSP-90 (heat shock protein 90) pathway (21, 22), as they need to resist apoptosis to protect themselves from their own proapoptotic SASP. These pathways represent a vulnerability of senescent cells and have paved the way for the identification of the first class of drugs capable of targeting them (senolytics), as described later.
We should also mention that although the SASP is a characteristic feature, not all senescent cells develop it (10).
Identifying the senescent state is useful not only to locate these cells but, more importantly, to develop targeted therapies and assess their effects on senescent cells or the SASP (Fig. 1). In further investigations, the phenotypic characteristics and molecular biomarkers of senescence have been discovered both in cultured cells and tissues. However, these markers are nonspecific, and senescent cells are heterogeneous. Therefore, the approach to detect senescent cells in vivo currently involves the combination of multiple methods, as summarized on table I (13). It remains to be determined which methods would be most efficient for future clinical practice.

Modified from: Farr JN, et al. Bone 2019 (13).
Figure 1. Inducers and mediators of senescence, SCAPs (anti-apoptotic pathways of senescent cells), SASP (senescence-associated secretory phenotype), and therapeutic targets of senotherapeutics on senescent cells.
Table I. Cellular senescence signaling at different levels.
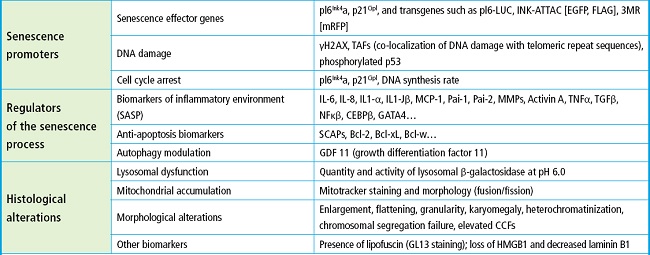
Bcl: B-cell Lymphoma; CCFs: cytoplasmic chromatin fragments; CEBP: CCAAT/enhancer-binding protein; GATA: guanine adenine thymine adenine; HMGB1: high mobility group box 1; H2AX: histone family member X; IL: interleukin; MCP1: monocyte chemoattractant protein-1; MMP: matrix metalloproteinase; NFκB: nuclear factor kappa B; SASP: senescence-associated secretory phenotype; SCAPs: anti-apoptotic pathways of senescent cells; TNF: tumor necrosis factor; Modified from: Farr JN, et al. (13,49,50).
One of the classic markers of senescence, traditionally used in vitro in skeletal tissues such as skin or adipose tissue, is the increased activity of the lysosomal enzyme β-galactosidase or senescence-associated β-galactosidase (SAβGal). The reason why this increased activity is detected in senescent cells is due to the high lysosomal content present in these cells (12).
ASSOCIATED DISEASES
Although senescent cells can appear at any stage of life, it is known that they accumulate in tissues as chronological age increases: adipose tissue, lung, skeletal muscle, heart, kidney, bone (13,17,19). As a matter of fact, the transplantation of small amounts of senescent cells around the knee joint in young mice induces a condition similar to osteoarthritis (23). Other conditions in which the accumulation of senescent cells has been demonstrated include progeroid syndromes in children, preeclampsia, age-related macular degeneration, liver cirrhosis, cancer, and vertebral spondylosis (4,10).
Similarly, recent studies have shown that senescent cells and the SASP play a prominent role in mediating age-related conditions such as cancer, osteoporosis, frailty, cardiovascular diseases, osteoarthritis, diabetes, and obesity, among others (3,6,16).
SENOLYTICS AND SENOMORPHICS
Given the potential of eliminating senescent cells or their proinflammatory secretion to treat age-related diseases and their consequences, the detection of senescent cells and the development of therapies targeting them have become important areas of research in the biomedical field. To this date, 2 main categories of drugs are being studied: senolytics, which specifically eliminate senescent cells, and senomorphics, which suppress the SASP without inducing cell death.
The first evidence supporting the hypothesis that treating senescent cells can alleviate age-related chronic diseases came from the creation of INK-ATTAC, a transgenic mouse model in which it is possible to identify (using p16Ink4a), isolate, and selectively eliminate senescent cells by administering a synthetic activating molecule: AP20187 (24). It was demonstrated that the elimination of p16Ink4a-positive senescent cells improved lifespan and had beneficial effects in multiple tissues (25).
Despite the promising nature of these findings, as it involves the insertion of a transgene, this genetic approach cannot be applied to humans (26). The first class of drugs that selectively eliminate senescent cells, senolytics, was then identified. These are small molecules that induce cell death by apoptosis specifically in senescent cells. They work by transiently disabling SCAPs, which, as mentioned earlier, protect senescent cells from their own proapoptotic SASP. The first senolytic compounds described, first in vitro and then in vivo, were dasatinib (D) and quercetin (Q), used together (D + Q). Dasatinib is a tyrosine kinase inhibitor, and quercetin is a flavonoid found in fruits and vegetables that inhibits tumor necrosis factor alpha (TNFα) (21).
In preclinical models, senolytics have been shown to delay, prevent, and/or alleviate frailty, cancer, and cardiovascular, hepatic, musculoskeletal, and neurological disorders. Initial trials suggest that they reduce senescent cell burden, decrease inflammation, and alleviate frailty in humans. Numerous clinical trials are currently underway for various diseases to safely translate these findings into the routine clinical practice in the management of age-related degenerative diseases (10,16).
We should mention that due to the beneficial functions of senescent cells, operating on the mechanisms through which a cell becomes senescent could have detrimental effects, such as an increased risk of cancer (27-30). Therefore, the goal is to target already formed senescent cells that accumulate damage and cause tissue dysfunction through their proinflammatory SASP. The following are the most important characteristics of each group.
As mentioned before, senescent cells resist apoptosis through their SCAPs. Senolytics act by transiently disabling these SCAPs, thus leading to cell death.
If we classify senolytic agents based on the anti-apoptotic pathways they target, as shown on figure 2, they can be divided into the following categories (16,22):
– BCL-2/BCL-W/BCL-XL pathway: Navitoclax, fisetin, A1331852, A1155463.
– PI3K/Akt pathway: quercetin, fisetin, piperlongumine.
– p53/p21/serpine pathway: quercetin, fisetin, FOXO4-related peptide.
– Ephrin/dependency receptor/tyrosine kinase pathway: dasatinib (eph receptor), piperlongumine (androgen receptors).
– HIF-1α pathway: quercetin, fisetin.
– HSP-90 pathway: tanespimycin, alvespimycin (this pathway was discovered later) (31).
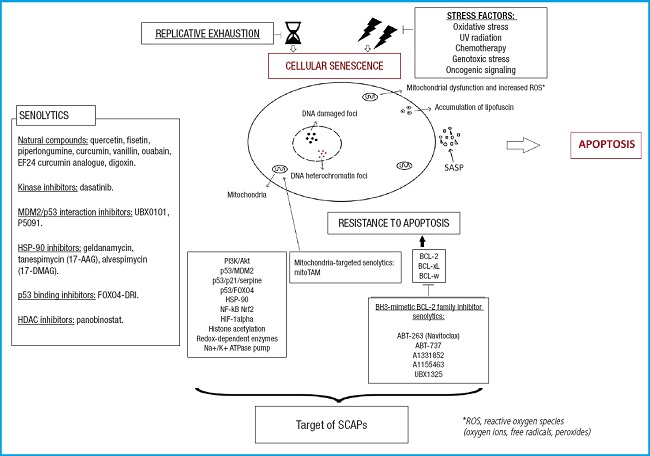
Modified from Lagoumtzi, et al. Free Radic Biol Med 2021 (16).
Figure 2. Main pathways targeted by senolytics. These include the BCL-2 family pathway, PI3K/Akt pathway, p53/p21/serpine pathway, ephrin/dependence receptor/tyrosine kinase pathway, and HIF-1α pathway.
Recently, it has been discovered that mitoTAM (mitochondria-targeted tamoxifen) acts as a senolytic drug, and it is postulated to be part of a new group of agents targeting mitochondria, which is still not fully understood (16).
Among the first batch of senolytics discovered (10), using the aforementioned approach, we find the following ones: dasatinib, quercetin (21), fisetin (32,33), luteolin, curcumin, curcumin analog EF24, navitoclax (ABT263) (34,35), A1331852, A1155463, geldanamycin, tanespimycin, alvespimycin, piperlongumine (36), FOXO4-related peptide (37), nutlin3a, ouabain, and proscurcinidin. Some of them are natural compounds, while others are synthetic small molecules (22).
Currently, methods for identifying senolytic drugs include random screenings of drug libraries, the use of nanotechnology, or immunomodulators (10).
From the perspective of finding drugs that are as specific as possible, it is interesting that they can target multiple pathways, which reduces the chances of off-target effects. A specific case that illustrates this issue is Navitoclax (34,35), that acts on a restricted range of senescent cells but has apoptotic effects on non-senescent cells. Therefore, its clinical use is limited due to side effects such as severe thrombocytopenia and neutropenia (30).
The SCAPs necessary to survive apoptosis differ among different types of senescent cells, making it challenging to find a single senolytic that is effective against all of them (4,22,30).
Senolytics can act synergistically (4,22), as is the case with D + Q, thus opening up the possibility of expanding their spectrum of action by combining different molecules, similar to antibiotics.
Since senescent cells take time to accumulate again in a tissue and acquire a SASP, administering senolytic drugs intermittently may be enough to achieve the therapeutic goals intended, thus minimizing side effects and the risk of effects beyond the intended site of action, and allowing administration during periods of good health (21). Additionally, since senescent cells do not divide, it is unlikely that these drugs will generate resistance. Regarding dosage, it will likely depend on the circumstance leading to senescence, which will determine a specific accumulation rate for each process (4,22,38).
Prior to clinical trials in humans to demonstrate the effectiveness of a senolytic drug, it is important to make sure that the effects seen are due to its intended action and not to off-target effects. Modified Koch's postulates (4) have been proposed and are fulfilled in mice treated with D + Q for various diseases. There are also indications of their fulfillment for fisetin, although there are more doubts surrounding Navitoclax (10).
As mentioned earlier, regarding the treatment of senescence, there is another category of drugs called senomorphic or senomodulating agents, which are small molecules that act indirectly on senescent cells by inhibiting their inflammatory SASP.
Some of these agents include resveratrol, apigenin, kaempferol, metformin, glucocorticoids, rapamycin, everolimus, ruxolitinib, or EGCG (epigallocatechin gallate). Among them, some are natural compounds, while others are approved drugs for specific indications by regulatory agencies. However, it remains to be seen whether dosages would be similar. Additionally, there are molecules identified as potential senomorphic agents, such as loperamide (4,16).
The targets of these drugs are the pathways through which the SASP is expressed or acts (Fig. 3). The most important pathways, along with some notable agents that act on them, are (16):
– Inhibition of NFκB pathway: resveratrol, apigenin, kaempferol, metformin, glucocorticoids.
– Inhibition of mTOR pathway: rapamycin, everolimus.
– JAK (janus kinase)/STAT pathway (JAK inhibition): ruxolitinib.
– Antibodies against the activity and function of specific SASP mediators such as IL-6 or IL-8.
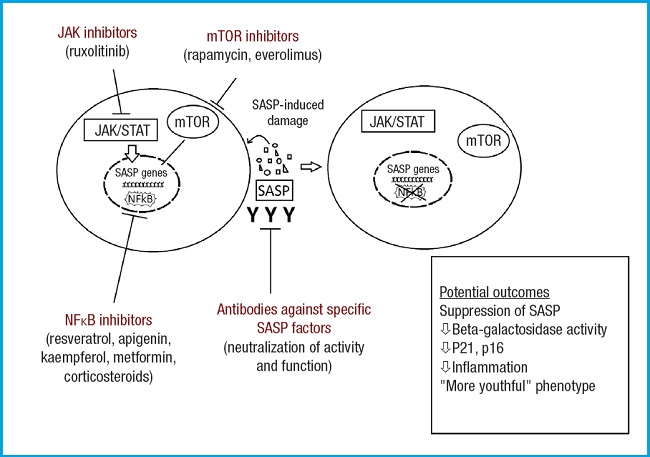
Modified from Lagoumtzi, et al. Free Radic Biol Med 2021 (16).
Figure 3. Main pathways targeted by senomorphics. These include the NFκB inhibition pathway, mTOR inhibition pathway, JAK/STAT pathway, and antibodies against specific SASP mediators. The mTOR kinase is key in cellular metabolism, controlling cellular catabolism and anabolism, determining whether cells (particularly cancer cells) should grow and proliferate. Additionally, mTOR has effects on apoptosis regulation. NFκB is widely used by eukaryotic cells as a regulator of genes that control cell proliferation and survival. The JAK/STAT signaling pathway is involved in processes such as immunity, cell division, cell death, and tumor formation.
BONE SENESCENCE
The role of senescence in age-related bone loss has recently been the subject of numerous investigations. With aging, bone remodeling is disrupted, thus leading to an imbalance between the amount of new bone formation and bone resorption, resulting in a negative balance that, over time, leads to osteoporosis.
Aging manifests in the bone as a decrease in bone tissue itself and an increased marrow fat. It is not clear whether marrow fat has a direct negative effect on bone formation, although it is likely because mesenchymal stem cells (MSC) are precursors to both marrow adipocytes and osteoblasts, thus suggesting a shift towards the adipogenic lineage that, along with adipokines, may contribute to the described situation.
While the exact processes thar cause these age-related changes are still not fully understood, it has been hypothesized that the basic mechanisms of aging including senescence, are responsible for age-related bone dysfunction (13).
For years, it has been impossible to demonstrate that senescent cells accumulate in bone with aging, as seen in other tissues. It was also unknown which cells in the bone microenvironment become senescent with age and whether they are capable of generating SASP (4). In the first study that addressed these issues (39), senescence and SASP markers were measured in vivo in young mice (both male and female) and compared to old mice, in highly enriched populations of various cell lineages. It was discovered that the expression of p16Ink4a consistently increased with aging in B cells, T cells, myeloid cells, osteoprogenitors, osteoblasts, and osteocytes. Additionally, it was found that p21Cip1 levels increased with aging in isolated cells from males enriched with osteocytes (while no change was seen in females). These findings in mice were validated in humans through the obtention of bone biopsies from elderly and young women, which revealed an age-related increase in both p16Ink4a and p21Cip1. Finally, it was demonstrated that SASP is mainly produced by senescent osteoprogenitors, senescent myeloid cells, and senescent osteocytes.
Although there are still significant gaps in knowledge and many unanswered questions, cellular senescence appears to be a global characteristic of natural aging in the bone, as in the rest of the body. This opens up the possibility that targeting senescence specifically could reduce the impact of a disease such as osteoporosis.
To determine if cellular senescence plays a role in age-related bone loss, 3 strategies were used: a genetic approach, a pharmacological approach to eliminate senescent cells (INKATTAC or senolytics, D + Q), and a senomorphic approach (using a JAK inhibitor, ruxolitinib) to inhibit SASP (26). All 3 interventions demonstrated prevention of bone loss in old mice, and none had effects on bone parameters in young mice, which is indicative of their specificity for aging (25).
In conclusion, studies on senescence in bone demonstrate that with aging, cells in the bone microenvironment (at least a subset of most cell types) become senescent and develop a heterogeneous SASP. Furthermore, they establish that senescent cells play a causal role in age-related bone loss, which can be alleviated in old mice by reducing the genetic or pharmacological burden with the first class of senolytics or with a senomorphic approach. This could represent a new strategy for treating or preventing osteoporosis, with potential advantages over conventional therapy (25,26).
Bisphosphonates are drugs commonly used in clinical practice to treat osteoporosis. One of them, zoledronic acid, could be considered a senotherapeutic agent. Animal experiments have shown that zoledronic acid extends cellular survival and delays senescence of mesenchymal stem cells, improves DNA repair by inhibiting the mevalonate and mTOR pathways, improves intestinal epithelial dysplasia, and prevents radiation-induced mutations (41). In 2007, an effect on the reduction of mortality regardless of the effect on the rate of fracture was observed. Since then, clinical trials, meta-analyses, and observational studies specifically designed to measure this relationship suggest that bisphosphonates (particularly nitrogen-containing ones including zoledronic acid) provide survival benefits in osteoporotic/osteopenic patients, as well as in patients previously exposed to various circumstances in intensive care, with cancer, or with heart disease (43). Recently, in women in their seventh decade of life with osteopenia, the administration of 5mg of IV zoledronic acid every 18 months showed a clear trend towards lower rates of mortality, cancer, and cardiovascular events (44), indicative of a potential systemic senotherapeutic effect.
As a matter of fact, a review article on the effects of bisphosphonates and lifespan shows studies in which bisphosphonates exhibit a protective effect with hazard ratios ranging from 0.56 to 0.94 (43).
Although further studies are needed to confirm these effects, the results obtained so far are extremely promising in the sense that drugs already used and useful in the treatment of a prevalent disease like osteoporosis, such as zoledronic acid, may have additional benefits similar to those described. Furthermore, understanding this association of zoledronic acid with increased survival opens the door to investigating the behavior of senescent cell markers in patients receiving zoledronic acid treatment and patients from other therapeutic groups, to identify surrogate markers of survival that would simplify future studies in this field.
CONCLUSIONS
Cellular senescence is a phenomenon involved in aging and chronic diseases associated with aging including osteoporosis. Its mechanism is complex and ambivalent: while it can be beneficial in certain contexts, especially in young tissues, it can be detrimental due to accumulation and its senescence-associated secretory phenotype (SASP) in other cases, leading to tissue dysfunction or exacerbating it.
To mitigate its role in the development of age-related diseases, therapies targeting senescent cells or their SASP are being investigated. A more comprehensive study of senescence markers would allow us to study the effect of certain drugs already used clinically for other indications and with lower risk compared to novel drugs.
ACKNOWLEDGEMENTS
The authors would like to thank the support given by Instituto de Salud Carlos III RICORS2040 (Renal Disease), the European Regional Development Fund (ERDF), and the Science, Technology, and Innovation Plan 2013-2017 and 2018-2022 of the Principality of Asturias, Spain (IDI-2021-000080).
REFERENCES
1. Seals DR, Justice JN, LaRocca TJ. Physiological geroscience: targeting function to increase healthspan and achieve optimal longevity. J Physiol 2016;594(8):2001-2024. DOI: 10.1113/jphysiol.2014.282665 [ Links ]
2. United Nations. Envejecimiento [Internet]. Naciones Unidas. 2019 [citado 20 de marzo de 2022]. Disponible en: https://www.un.org/es/global-issues/ageing [ Links ]
3. Song S, Lam EW, Tchkonia T, Kirkland JL, Sun Y. Senescent Cells: Emerging Targets for Human Aging and Age-Related Diseases. Trends Biochem Sci 2020;45(7):578-92. DOI: 10.1016/j.tibs.2020.03.008 [ Links ]
4. Khosla S, Farr JN, Tchkonia T, Kirkland JL. The role of cellular senescence in ageing and endocrine disease. Nat Rev Endocrinol 2020;16(5):263-75. DOI: 10.1038/s41574-020-0335-y [ Links ]
5. Tchkonia T, Zhu Y, van Deursen J, Campisi J, Kirkland JL. Cellular senescence and the senescent secretory phenotype: therapeutic opportunities. J Clin Invest 2013;123(3):966-72. DOI: 10.1172/JCI64098 [ Links ]
6. Kaur J, Farr JN. Cellular senescence in age-related disorders. Transl Res 2020;226:96104. DOI: 10.1016/j.trsl.2020.06.007 [ Links ]
7. Kirkland JL, Stout MB, Sierra F. Resilience in Aging Mice. J Gerontol A Biol Sci Med Sci 2016;71(11):1407-14. DOI: 10.1093/gerona/glw086 [ Links ]
8. Kennedy BK, Berger SL, Brunet A, Campisi J, Cuervo AM, Epel ES, et al. Geroscience: linking aging to chronic disease. Cell 2014;159(4):709-13. DOI: 10.1016/j.cell.2014.10.039 [ Links ]
9. López-Otín C, Blasco MA, Partridge L, Serrano M, Kroemer G. The hallmarks of aging. Cell 2013;153(6):1194-217. DOI: 10.1016/j.cell.2013.05.039 [ Links ]
10. Kirkland JL, Tchkonia T. Senolytic drugs: from discovery to translation. J Intern Med 2020;288(5):518-36. DOI: 10.1111/joim.13141 [ Links ]
11. Hayflick L, Moorhead PS. The serial cultivation of human diploid cell strains. Exp Cell Res 1961;25:585-621. DOI: 10.1016/0014-4827(61)90192-6 [ Links ]
12. Galiana Guillem I, Martínez Máñez R (dir), Orzáez Calatayud M (dir). Desarrollo de nuevos nanodispositivos terapéuticos aplicados al tratamiento de enfermedades relacionadas con procesos de senescencia [tesis doctoral en Internet]. [Valencia]: Universitat Politèctica de València; 2020 [citado 15 marzo de 2022]. Disponible en: http://hdl.handle.net/10251/151950 [ Links ]
13. Farr JN, Khosla S. Cellular senescence in bone. Bone. 2019;121:121-33. DOI: 10.1016/j.bone.2019.01.015 [ Links ]
14. Hernandez-Segura A, Nehme J, Demaria M. Hallmarks of Cellular Senescence. Trends Cell Biol 2018;28(6):436-53. DOI: 10.1016/j.tcb.2018.02.001 [ Links ]
15. Demaria M, Ohtani N, Youssef SA, Rodier F, Toussaint W, Mitchell JR, et al. An essential role for senescent cells in optimal wound healing through secretion of PDGF-AA. Dev Cell 2014;31(6):722-33. DOI: 10.1016/j.devcel.2014.11.012 [ Links ]
16. Lagoumtzi SM, Chondrogianni N. Senolytics and senomorphics: Natural and synthetic therapeutics in the treatment of aging and chronic diseases. Free Radic Biol Med 2021;171:169-90. DOI: 10.1016/j.freeradbiomed.2021.05.003 [ Links ]
17. Tchkonia T, Palmer AK, Kirkland JL. New Horizons: Novel Approaches to Enhance Healthspan Through Targeting Cellular Senescence and Related Aging Mechanisms. J Clin Endocrinol Metab 2021;106(3):e1481-e1487. DOI: 10.1210/clinem/dgaa728 [ Links ]
18. DeVito LM, Barzilai N, Cuervo AM, Niedernhofer LJ, Milman S, Levine M, et al. Extending human healthspan and longevity: a symposium report. Ann N Y Acad Sci 2022;1507(1):70-83. DOI: 10.1111/nyas.14681 [ Links ]
19. Kirkland JL, Tchkonia T. Cellular Senescence: A Translational Perspective. EBioMedicine 2017;21:21-8. DOI: 10.1016/j.ebiom.2017.04.013 [ Links ]
20. Gorgoulis V, Adams PD, Alimonti A, Bennett DC, Bischof O, Bishop C, et al. Cellular Senescence: Defining a Path Forward. Cell 2019;179(4):813-27. DOI: 10.1016/j.cell.2019.10.005 [ Links ]
21. Zhu Y, Tchkonia T, Pirtskhalava T, Gower AC, Ding H, Giorgadze N, et al. The Achilles'heel of senescent cells: from transcriptome to senolytic drugs. Aging Cell 2015;14(4):644-58. DOI: 10.1111/acel.12344 [ Links ]
22. Kirkland JL, Tchkonia T, Zhu Y, Niedernhofer LJ, Robbins PD. The Clinical Potential of Senolytic Drugs. J Am Geriatr Soc 2017;65(10):2297-301. DOI: 10.1111/jgs.14969 [ Links ]
23. Xu M, Bradley EW, Weivoda MM, Hwang SM, Pirtskhalava T, Decklever T, et al. Transplanted Senescent Cells Induce an Osteoarthritis-Like Condition in Mice. J Gerontol A Biol Sci Med Sci 2017;72(6):780-5. [ Links ]
24. Baker DJ, Wijshake T, Tchkonia T, LeBrasseur NK, Childs BG, van de Sluis B, et al. Clearance of p16lnk4a-positive senescent cell delays ageing-associated disorders. Nature 2011;479(7372):232-6. DOI: 10.1038/nature10600 [ Links ]
25. Khosla S, Farr JN, Kirkland JL. Inhibiting Cellular Senescence: A New Therapeutic Paradigm for Age-Related Osteoporosis. J Clin Endocrinol Metab 2018;103(4):12821290. DOI: 10.1210/jc.2017-02694 [ Links ]
26. Farr JN, Xu M, Weivoda MM, Monroe DG, Fraser DG, Onken JL, et al. Targeting cellular senescence prevents age-related bone loss in mice. Nat Med 2017;23(9):1072-9. DOI: 10.1038/nm.4385 [ Links ]
27. Takeuchi S, Takahashi A, Motoi N, Yoshimoto S, Tajima T, Yamakoshi K, et al. Intrinsic cooperation between p16Ink4a and p21Waf1/Cip1 in the onset of cellular senescence and tumor suppression in vivo. Cancer Res 2010;70(22):9381-90. DOI: 10.1158/0008-5472.CAN-10-0801 [ Links ]
28. Larsen CJ. PRB, p53, p16Ink4a/ARF, sénescence cellulaire et transformation maligne [pRB, p53, p16Ink4a, senescence and malignant transformation]. Bull Cancer 2004;91(5):399-402. French. [ Links ]
29. Faget DV, Ren Q, Stewart SA. Unmasking senescence: context-dependent effects of SASP in cancer. Nat Rev Cancer 2019;19(8):439-53. DOI: 10.1038/s41568-019-0156-2 [ Links ]
30. Kang C. Senolytics and Senostatics: A Two-Pronged Approach to Target Cellular Senescence for Delaying Aging and Age-Related Diseases. Mol Cells 2019;42(12):821827. [ Links ]
31. Fuhrmann-Stroissnigg H, Ling YY, Zhao J, McGowan SJ, Zhu Y, Brooks RW, et al. Identification of HSP90 inhibitors as a novel class of senolytics. Nat Commun 2017;8(1):422. DOI: 10.1038/s41467-017-00314-z [ Links ]
32. Zhu Y, Doornebal EJ, Pirtskhalava T, Giorgadze N, Wentworth M, Fuhrmann-Stroissnigg H, et al. New agents that target senescent cells: the flavone, fisetin, and the BCL-XL inhibitors, A1331852 and A1155463. Aging (Albany NY) 2017;9(3):955-63. DOI: 10.18632/aging.101202 [ Links ]
33. Yousefzadeh MJ, Zhu Y, McGowan SJ, Angelini L, Fuhrmann-Stroissnigg H, Xu M, et al. Fisetin is a serotherapeutic that extends health and lifespan. EBioMedicine 2018;36:18-28. DOI: 10.1016/j.ebiom.2018.09.015 [ Links ]
34. Zhu Y, Tchkonia T, Fuhrmann-Stroissnigg H, Dai HM, Ling YY, Stout MB, et al. Identification of a novel senolytic agent, navitoclax, targeting the Bcl-2 family of antiapoptotic factors. Aging Cell 2016;15(3):428-35. DOI: 10.1111/acel.12445 [ Links ]
35. Chang J, Wang Y, Shao L, Laberge RM, Demaria M, Campisi J, et al. Clearance of senescent cells by ABT263 rejuvenates aged hematopoietic stem cells in mice. Nat Med 2016;22(1):78-83. DOI: 10.1038/nm.4010 [ Links ]
36. Wang Y, Chang J, Liu X, Zhang X, Zhang S, Zhang X, et al. Discovery of piperlongumine as a potential novel lead for the development of senolytic agents. Aging (Albany NY) 2016;8(11):2915-26. DOI: 10.18632/aging.101100 [ Links ]
37. Baar MP, Brandt R, Putavet DA, Klein J, Derks K, Bourgeois B, et al. Targeted Apoptosis of Senescent Cells Restores Tissue Homeostasis in Response to Chemotoxicity and Aging. Cell 2017;169(1):132-147.e16. DOI: 10.1016/j.cell.2017.02.031 [ Links ]
38. Palmer AK, Gustafson B, Kirkland JL, Smith U. Cellular senescence: at the nexus between ageing and diabetes. Diabetología 2019;62(10):1835-41. DOI: 10.1007/s00125-019-4934-x [ Links ]
39. Farr JN, Fraser DG, Wang H, Jaehn K, Ogrodnik MB, Weivoda MM, et al. Identification of Senescent Cells in the Bone Microenvironment. J Bone Miner Res 2016;31(11):19201929. DOI: 10.1002/jbmr.2892 [ Links ]
40. Xu M, Tchkonia T, Ding H, Ogrodnik M, Lubbers ER, Pirtskhalava T, et al. JAK inhibition alleviates the cellular senescence-associated secretory phenotype and frailty in old age. Proc Natl Acad Sci U S A 2015;112(46):E6301-E6310. DOI: 10.1073/pnas.1515386112 [ Links ]
41. Misra J, Mohanty ST, Madan S, Fernandes JA, Hal Ebetino F, Russell RG, et al. Zoledronate Attenuates Accumulation of DNA Damage in Mesenchymal Stem Cells and Protects Their Function. Stem Cells 2016;34(3):756-67. DOI: 10.1002/stem.2255 [ Links ]
42. Lyles KW, Colón-Emeric CS, Magaziner JS, Adachi JD, Pieper CF, Mautalen C, et al;HORIZON Recurrent Fracture Trial. Zoledronic acid and clinical fractures and mortality after hip fracture. N Engl J Med 2007;357(18):1799-809. DOI: 10.1056/NEJMoa074941 [ Links ]
43. Center JR, Lyles KW, Bliuc D. Bisphosphonates, and lifespan. Bone 2020;141:115566. DOI: 10.1016/j.bone.2020.115566 [ Links ]
44. Reid IR, Horne AM, Milhov B, Stewart A, Garratt E, Bastin S, et al. Effects of Zoledronate on Cancer, Cardiac Events, and Mortality in Osteopenic Older Women. J Bone Miner Res 2020;35(1):20-7. DOI: 10.1002/jbmr.3860 [ Links ]
45. Marie PJ. Bone cell senescence: mechanisms and perspectives. J Bone Miner Res 2014;29(6):1311-21. DOI: 10.1002/jbmr.2190 [ Links ]
46. Marie PJ, Cohen-Solal M. The Expanding Life and Functions of Osteogenic cells: from Simple Bone-Making Cells to Multifunctional Cells and Beyond. J Bone Miner Res 2018;33(2):199-210. DOI: 10.1002/jbmr.3356 [ Links ]
47. Reid IR, Horne AM, Mihov B, Stewart A, Garratt E, Wong S, et al. Fracture Prevention with Zoledronate in Older Women with Osteopenia. N Engl J Med 2018;379(25):24072416. DOI: 10.1056/NEJMoa1808082 [ Links ]
48. Xu M, Pirtskhalava T, Farr JN, Weigand BM, Palmer AK, Weivoda MM, et al. Senolytics improve physical function and increase lifespan in old age. Nat Med 2018;24(8):12461256. DOI: 10.1038/s41591-018-0092-9 [ Links ]
49. González-Gualda E, Baker A G, Fruk L, Muñoz-Espín D. A guide to assessing cellular senescence in vitro and in vivo. FEBS J 2021;288(2):56-80. DOI: 10.1111/febs.15570 [ Links ]
50. Sun J, Li Y, Yang X, Dong W, Yang J, Hu Q, et al. Growth differentiation factor 11 accelerates liver senescence through the inhibition of autophagy. Aging Cell 2022;21:e13532. DOI: 10.1111/acel.13532 [ Links ]
Pena Larrea L, de Blas Rodríguez M, Naves Díaz M, Gómez Alonso C. Cellular senescence as a pathogenic factor and potential therapeutic target in osteoporosis. Rev Osteoporos Metab Miner 2023;15(3):115-124
The authors would like to thank the support given by Instituto de Salud Carlos III RICORS2040 (Renal Disease), the European Regional Development Fund (ERDF), and the Science, Technology, and Innovation Plan 2013-2017 and 2018-2022 of the Principality of Asturias, Spain (IDI-2021-000080).
Received: April 18, 2023; Accepted: May 25, 2023