Introduction
The eye is responsible for vision and is one of the most complex and sophisticated sense organs. Its anatomical structure and physiology is especially adapted to allow the passage of light and to provide protection against external agents1. At the anatomical level, it is divided into the anterior and posterior segments, which constitute one-third and two-thirds of the total dimension of the eye, respectively. The anterior segment includes the lacrimal apparatus, cornea, conjunctiva, anterior and posterior chambers, iris, ciliary bodies, lens, and aqueous humor; and the posterior segment consists of the sclera, choroid, retina, Bruch's membrane, vitreous humor, optic nerve, and retinal blood vessels2. Its different components make it operate as a complex system with numerous barriers that limit drug penetration within the organ3.
Ocular drug administration plays an important role in the treatment of common ocular diseases such as glaucoma, macular degeneration, diabetic retinopathy, infections (conjunctivitis, keratitis, endophthalmitis, etc.), and autoimmune disorders (Sjögren syndrome, uveitis, etc.). Each of the ocular administration routes (topical, intravitreal, and periocular) has advantages and disadvantages, however, a key factor common to all these routes is low drug bioavailability at the target site4,5. Therefore, new ocular delivery systems need to be developed such that drugs can be made available at the target site in sufficient concentrations over a precise period of time to produce the desired effect.
The objective of this article is to review current drug delivery systems and those in development for each of the drug administration routes used in the treatment of eye disease.
We conducted an initial search of PubMed, Medline, Cochrane database, and Google Scholar including publications in English and Spanish. Various literature search strategies were conducted combining key terms and Medical Subject Headings (MeSH) to ensure that all relevant papers were found: “ocular drug delivery”, “ophthalmic drug delivery”, “intraocular drug delivery”, “intravitreal delivery”, “ocular inserts”, and “intraocular devices”. Selected articles included original papers and review articles. The ClinicalTrials database was also consulted for additional information on clinical trials related to new forms of ocular administration (https://clinicaltrials.gov)).
Physiological barriers
Tear film
The tear layer is a thin liquid film (3 μm) covering the corneal and conjunctival surfaces. It has different functions, among which its lubricant, nutritional, and antibacterial activity are of special relevance. Under normal conditions, its volume ranges between 8 μL and 10 μL although this can be exceeded in certain physiological and pathological situations. It consists of the lipid, aqueous, and mucin layers2. The outermost layer is the lipid layer, whose main function is to retard evaporation from the aqueous layer6); the middle layer is the aqueous layer, constituting around 90% of the total volume of tears; and the innermost layer is the mucin layer, constituted by glycoproteins which, due to their hydrophilicity, contribute to the adequate wetting of the cornea and conjunctiva7. Different protein compounds are also present, one of the most relevant is lysozyme, which has antimicrobial activity8.
Tears are the main barrier to the absorption of topically administered drugs. Drainage through the nasolacrimal duct, protein binding with drug molecules, and continuous tear turnover (1 mL/min) drastically reduce effective drug concentrations at the target site9,10.
Cornea
The cornea is a transparent avascular connective tissue that acts as the first structural barrier of the eye. Together with the tear film, it provides an adequate refractive surface that confers optical functionality11. The cornea is the main absorption route of topically administered ocular drugs. It is made up of six different layers: epithelium, Bowman's membrane, stroma, Dua's layer, Descemet's membrane, and endothelium. The outer layer prevents absorption, especially that of hydrophilic substances. Next, the stroma comprises keratocytes and connective tissue, forms 90% of the thickness of the cornea, and constitutes the largest reservoir for hydrophilic substanCes9,12. The inner layer allows the passage of substances with relative ease, including high molecular weight proteins. Due to the nature of the different layers, drug absorption at the corneal level is fundamentally affected by their hydrophilic-lipophilic balance. However, the presence of erosions and ulcers implies greater drug penetration13.
Blood-ocular barriers
Blood-ocular barriers protect the eye from circulating substances in the blood and thus prevent many systemically administered drugs from entering the eye. There are two types of blood-ocular barrier: the blood-aqueous barrier, which is formed by the epithelium of the ciliary bodies and protects the anterior segment; and the blood-retinal barrier, which protects the posterior segment and controls the entry of drugs into the vitreous cavity from the systemic circulation14,15.
These barriers prevents drug access at the intraocular level, although some factors such as inflammation or edema can alter them and make drug concentrations at this level higher than expected16,17.
Administration routes and drug delivery systems
The drug administration route is a key aspect of all ocular therapies. The topical route is the most frequently used18. Drugs administered by this route must meet a set of requirements. Tear fluid has a pH of between 7.4 and 7.7 and may be modified due to eye diseases. Eye drop pH has to be adapted to this range to avoid sensations such as pain, irritation, and tearing, although solutions with a pH other than physiological pH are sometimes used to optimize stability, solubility, and the degree of dissociation of the active principle. The buffering power of carbonic acid, weak organic acids, and proteins present in tear fluid relatively quickly neutralize solutions with a wide pH range (3.5-10.5) providing they are not buffered. The further the pH of the administered solution from the physiological pH of tears, the longer it takes to achieve neutralization19.
Sterility is one of the most important requirements because an association has been found between the use of contaminated preparations and different types of eye infections20. Clarity is also essential because suspended particles can cause corneal abrasions. The average particle size in most ophthalmic suspensions is less than 10 µm21. Finally, the osmolarity should be similar to that of the tear fluid (300.5 ± 7.2 mOsm/kg), although the eye can accept a certain range of osmotic pressure without irritation (osmolarity equivalent to sodium chloride solutions between 0.5% and 1.8%). Generally, slightly hypertonic mixtures are better tolerated than hypotonic ones, but they have the drawback that they cause the osmotic movement of water towards the conjunctival sac, causing dilution of the instilled drug19,20,22.
Drug delivery systems to the anterior segment
After topical drug administration, absorption takes place either through the corneal route or the noncorneal route. Drugs with high corneal permeability, such as water-soluble small molecules (i.e. with molecular weights less than 100 Da23), follow the corneal route15,24. Such drugs pass through the epithelium, stroma, and endothelium by passive diffusion into the anterior chamber, where they perform their pharmacological function25-27or bind to melanin (iris and ciliary body)13 or plasma proteins28. Any remaining drug and metabolites are eliminated through the trabecular meshwork through Schlemm's canal to the systemic blood circulation (conventional route) or through the iris to the uveoscleral outflow pathway (nonconventional route) and thence to the systemic blood circulation29-32. A smaller proportion of the drug reaches the posterior chamber through the iris by diffusion through the aqueous humor outflow pathway. As a result, vitreal drug concentrations are 10 to 100 times lower than in the aqueous humor and the cornea, respectively15. All these dynamics can be altered by eye movements33,34 or ophthalmic diseases25,35,36.
Drugs with low corneal permeability (high molecular weight molecules) enter the eye through the conjunctiva or the sclera following the non-corneal route30,37-40. This route is relevant in the case of small moderately lipophilic molecules such as timolol41. In general, the noncorneal route is of less importance although it can be artificially enhanced with the use of iontophoresis42. Drugs reach the vitreous humor through passive diffusion and active transport mechanisms. Once inside the vitreous chamber, the aqueous humor flow either transports the drug to the anterior chamber or it is eliminated by passive diffusion through the retinal pigment epithelium and retinal capillary endothelial cells (blood-retinal barrier) via the choroidal circulation towards the systemic blood circulation15,30,43. In comparison to the corneal absorption route, the noncorneal route provides a 20-fold lower drug concentration in the anterior chamber44.
Topical administration
Eye drops and ointments are the most widely used ophthalmic drug delivery systems (approximately 90%)18 due to their low production cost and ease of administration (Figure 1). Nevertheless, solutions have very low ocular bioavailability because of significant precorneal drug loss through tearing, blinking, systemic elimination, and tear renewal18. Frequent administrations are needed to maintain effective drug concentrations, leading to low therapeutic adherence and consequent therapeutic failure.
One of the most frequently used techniques to increase ocular surface contact time is the use of traditional viscous agents such as hydroxymethyl cellulose, polyvinyl alcohol, hydroxypropyl methylcellulose, polyethylene glycols, and polyvinylpyrrolidone. Natural polymers are also used such as hyaluronic acid, Veegum®, alginates, xanthan gum, gelatin, acacia, and tragacanth21,45.
Ophthalmic emulsions improve the solubility and bioavailability of insoluble drugs, provide sustained release, improve corneal absorption, and prolong precorneal residence time. In ophthalmology, o/w emulsions are preferred over w/o emulsions because of better tolerability and decreased ocular irritation due to the external aqueous pase46. Suspensions can be used as an alternative vehicle with low-solubility drugs, thus increasing the drug particle retention time in suspension as well as contact time. Table 1 shows some examples of ophthalmic emulsions and suspensions currently available on the market.
Table 1. Commercial presentations for the topical ophthalmic administration of sustained-release drugs
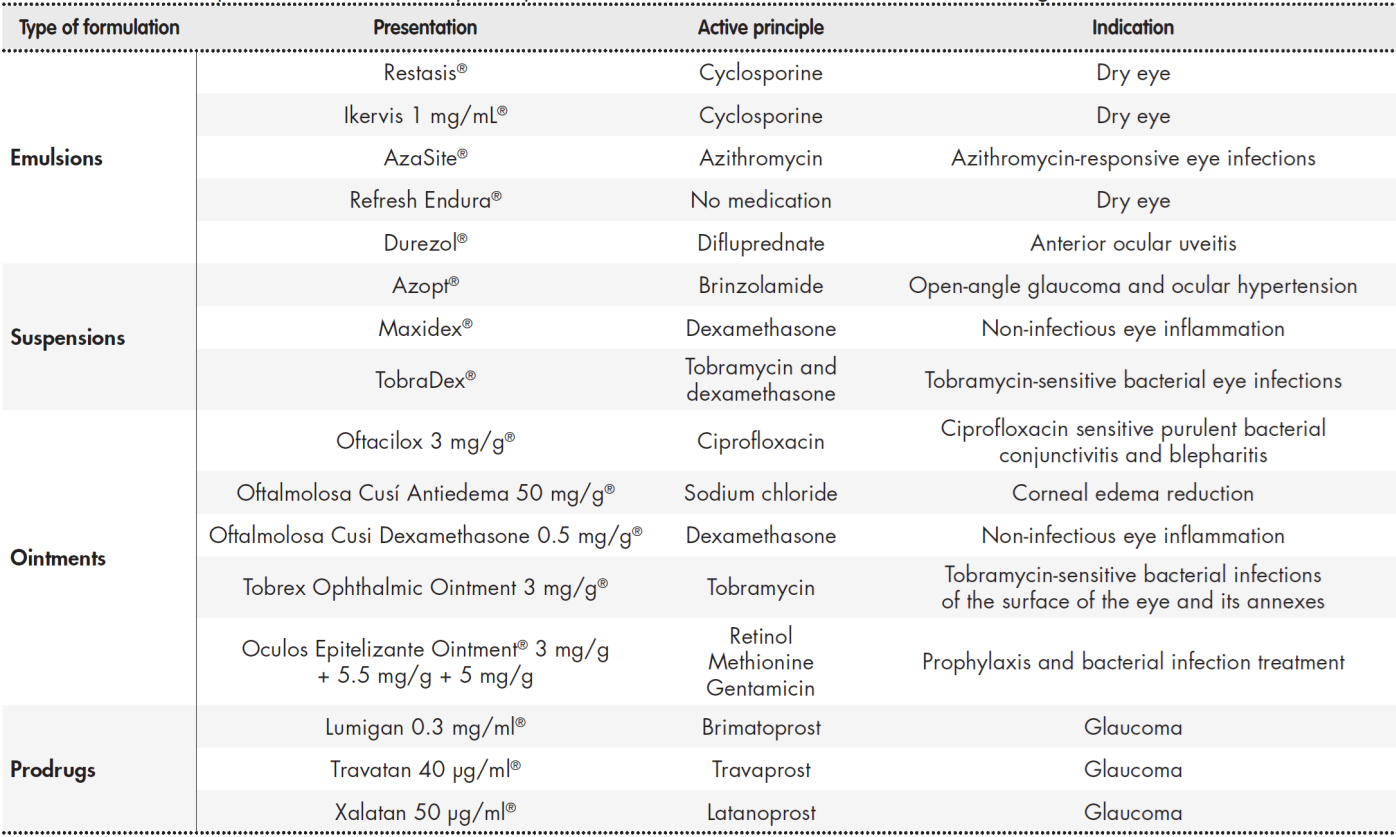
After solutions and suspensions, ophthalmic ointments are the most popular dosage forms. The vehicle/base for ophthalmic use must not cause eye discomfort and must be compatible with the rest of the formulation components. There is a wide variety of ophthalmic ointments available on the market, most of which have anti-infective, anti-edemic, or anti-inflammatory properties (Table 1). There has been a recent increase in the use of water-soluble bases known as gels because of their advantages over vaseline bases, such as better spreading capacity and their characteristics of pH, lubricity, stability, and low irritability. Polymers used for the preparation of gels generally include PEG 200, PEG 400, carboxymethyl cellulose, carbopol, methyl polymethacrylate, and Lutrol FC-127. These polymers also have mucoadhesive properties that improve drug contact time21. Recently, hospital pharmacy services have begun to include these kinds of ingredients in compounded preparations, such as the use of hyaluronic acid in artificial tears and carboxymethyl cellulose in the production of vancomycin and cysteamine eye drops47.
Another strategy is the use of prodrugs, such as the prostaglandin F2α analogues Bimatoprost (Lumigan®), Travaprost (Travatan®), and Latanoprost (Xalatan®), which are used to reduce intraocular pressure in glaucoma. Furthermore, the Food and Drug Administration (FDA) of the United States has authorized loteprednol etabonate (Lotemax®), a topical corticosteroid derived from the chemical structure of prednisolone, for the treatment of eye allergies and inflammations, although it is not available in the European market48.
Advances have recently been made in research on nanocarriers. These systems allow the administration of a wide variety of active ingredients (including macromolecules) providing stable conditions for labile compounds and better control of drug delivery, thus improving ocular bioavailability. There is a wide variety of components within this group such as polymeric nanoparticles, lipid nanoparticles, cyclodextrins, liposomes, niosomes, dendrimers, nanosuspensions, and emulsions21,42,49, all of which are currently under investigation. These types of systems could be used in the field of compounding, such as cyclodextrins50,51, which reduce the toxicity of active ingredients52 and enhance the solubility of molecules that are barely soluble in vehicles used for ophthalmic administration.
Contact lenses are medical devices that can be loaded with active ingredients, thereby acting as non-invasive drug-delivery systems and providing sustained release in tear fluid located between the cornea and the lens54. Generally, drugs and polymers used for these lenses have low affinity. Thus, to achieve controlled sustained release, a series of modifications are needed such as the incorporation of polymeric nanoparticles, microemulsions, micelles, liposomes, diffusion barriers (e.g. vitamin E) and so on, or the use of sophisticated loading techniques such as molecular imprinting, drug-loaded films, ion ligand polymeric systems, or supercritical fluid technology55,56. When such modifications are introduced, it is essential that aspects such as water content, mechanical properties, ionic permeability, transparency, and oxygen permeability remain unchanged. Up to the present, hindrances to their commercialization have included issues such as drug stability during processing/ manufacturing, achieving zero-order release kinetics, avoiding drug release during the post-manufacturing monomer extraction step, protein adherence, drug release during storage, and cost-benefits46. However, they have potential applicability in the field of ophthalmic compounding, because drugs and excipients can be easily loaded onto them under sterile conditions as part of a relatively simple low-cost formulation process. Thus, an ophthalmic drug delivery system could be made available that is easy and comfortable to administer to the patient and has a dosing schedule that leads to better adherence than that obtained under frequent instillation of ophthalmic drops. These aspects would be of interest in the setting of severe eye infections or rare diseases such as ocular cystinosis.
Inserts and implants in the anterior segment
The goal of ocular inserts and implants is to improve bioavailability and achieve sustained drug delivery. In order to administer medications to the anterior segment of the eye, these systems can be placed under the eyelid, at the bottom of the conjunctival sac, in the anterior chamber, in the subconjunctival space, or in the episcleral region (Figure 2). However, it is difficult to develop implants for the anterior chamber, because they can be susceptible to movement due to the low viscosity of the aqueous humor, causing irreversible damage to endothelial cells.
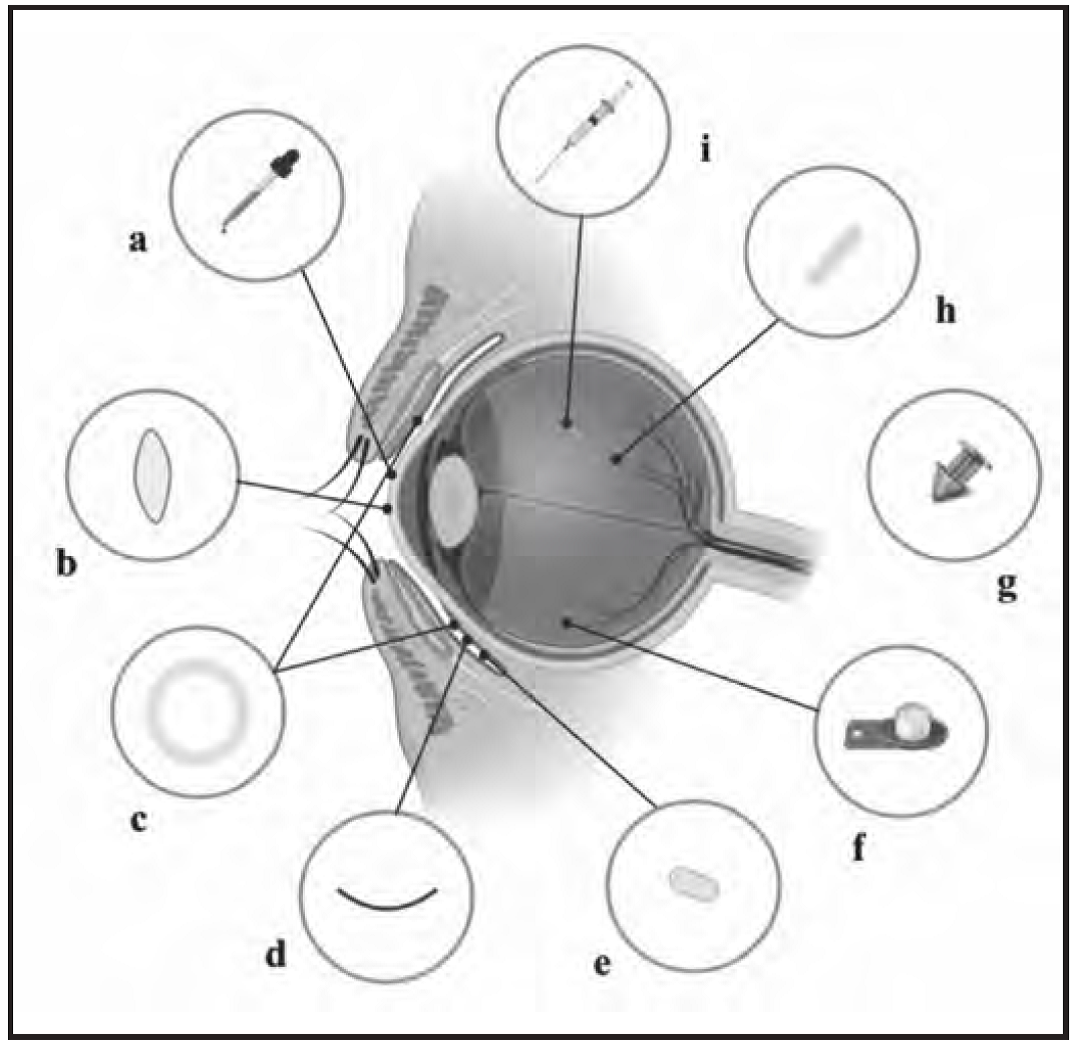
Figura 2. Overview of drug delivery devices. a) Eye drops. b) Drug-loaded contact lenses. c) Conjunctival insert, such as Ocusert®. d) The OphthaCoil® conjunctival insert: a device placed behind the lower eyelid in the fornix conjunctiva. e) Conjunctival tablet, Lacrisert® or Mydriasert®: these tablets are placed in the inferior conjunctival fornix. f) Retrisert intravitreal insert: implanted in the vitreous chamber and sewn to the sclera. g) Punctal plug, such as Dextenza or Evolute. h) Intravitreal insert, such as Illuven or Surodex®: implanted or injected into the vitreous chamber. i) Intravitreal injection.
These devices can be biodegradable or nonbiodegradable (Table 2). Biodegradable or resorbable devices are noninvasive and do not need to be removed. However, most of these devices have limited action time and therefore may require frequent administration. Furthermore, the complete material and its metabolites must not be toxic. Other challenges include the prevention of accidental device loss and increased tear production after placement, which increases the risk of massive drug reléase57. The Ocusert® system was the first commercially available ocular insert for the treatment of glaucoma. This ocular therapeutic system delivers a small amount of pilocarpine over one week. It consists of two ethylene-vinyl acetate copolymer membranes that provide zero-order kinetics58. This insert was withdrawn from the market in 1998 as a consequence of the adverse effects of pilocarpine, such as eyebrow pain and miosis18.
The only resorbable conjunctival device on the market is Lacrisert®, which is a small hydroxypropyl cellulose tablet placed in the inferior conjunctival fornix. It slowly dissolves and creates an artificial tear film to treat dry eye59. However, it can cause blurred vision, leading to device removal in 8.7% of patients60. Another cul-de-sac implant is DSP-Visulex, which has currently completed a phase-II clinical trial for the treatment of anterior uveitis with dexamethasone (NCT02309385)61.
Nonresorbable devices form another group. These include rod-shaped devices, which are placed in the superior or inferior conjunctival fornix to administer drugs through the noncorneal absorption route. Since 2004, Mydriasert® has been available on the European market. It is an ethylcellulose tablet loaded with tropicamide hydrochloride and phenylephrine to obtain mydriasis two hours before surgery. However, there are no significant differences in pupil dilation between topical mydriatic eye drops and Mydriasert®, although its dilation speed is slower62. Another type of device is OphthaCoil®, a spiral stainless steel wire, which is placed in the lower conjunctival sac. It can carry medications on its inner lumen (loaded in microspheres or filaments) or outside on the SlipSkin® lining. These devices have been tested with pradofloxacin and mydriatic agents (phenylephrine hydrochloride and tropicamide) in Beagle dogs63 and horses64.
The Helios™ device consists of a polypropylene support ring within a silicone matrix loaded with bimatoprost. The results of clinical trials showed that its placement in the eye reduced intraocular pressure in glaucoma patients over six months. However, no significant differences were found between this device and regular 0.5% preservative-free timolol ophthalmic solution. The dropout rate was higher among patients with the Helios device. The main adverse event was mucus discharge65.
Punctal plugs are another type of ocular device that have shown potential as drug delivery systems. These plugs are small biocompatible implants that were initially used to treat dry eye by inserting them into the tear ducts or to block tear drainage. They have the advantages of being non-invasive and maintaining the sustained release of the drug through diffusion. However, they have an increased risk of associated complications, such as conjunctivitis, corneal abrasion, tear duct obstruction, excessive tearing, and plug extrusion42. The materials used in their preparation include silicone, hydroxyethyl methacrylate, and polycaprolactone, but they require removal after the complete release of the active ingredient. Clinical trials are currently underway on the administration of latanoprost, bimatoprost, olopatadine, moxifloxacin, and cyclosporine A18. In 2018, the FDA authorized the marketing of Dextenza®, which contains dexamethasone 0.4 mg for the treatment of postoperative inflammation and pain. However, this format is not available in the European market.
The transfer of these types of devices and implants to the setting of ophthalmic compounding formulation is unlikely because of the complexity of their preparation and sterilization, which would require equipment not currently available in hospital pharmacy services.
Drug delivery systems to the posterior segment
In the field of ophthalmology, intravitreal administration has become one of the most widely performed procedures for the administration of anti-VEGF antibodies (bevacizumab, ranibizumab, aflibercept) in patients with age-related vascular degeneration (AMD)66.
Intravitreal injections
In intravitreal administration, the needle is inserted perpendicular to the sclera, between the horizontal and vertical rectus muscles, in order to release the drug in the vitreous chamber where it is distributed and reaches different targets in the posterior eye segment67 (Figure 1). Depending on the nature of the drug and the formulation used, the vitreous humor can act as an important barrier to the release of the drug or its influence may be insignificant15.
After intravitreal administration, drug concentrations at the blood-ocular barriers are clearly higher than those obtained after topical or systemic administration. At this level, drug clearance can occur through the anterior or posterior pathways. Any drug can pass through the anterior route by diffusion through the vitreous humor to the posterior chamber, where the aqueous humor is located, and then eliminated through the trabecular meshwork. However, the posterior route is only available to those drugs that are capable of crossing the endothelium and epithelium that form the blood-ocular barriers. Although these blood-ocular barriers do not allow the passage of proteins and large molecules, they do allow the passage of small molecules15. As a consequence, the clearance of small molecules from the vitreous is much faster than that of molecules with high molecular weights15,53,68.
A priority in AMD research is to decrease the number of visits to the ophthalmologist and the number of intravitreal injections administered69. In this sense, the need to develop new intravitreal drug delivery systems is based on the fact that reductions in the frequency of administration decreases the risk of associated adverse effects and optimizes healthcare costs5. Thus, the vast majority of intravitreal drug delivery systems have been studied using anti-VEGF drugs.
Ocular inserts in the posterior segment
There are various challenges related to the design of new intravitreal drug delivery systems. On the one hand, the size of these systems must be of the order of micrometers or nanometers to fit inside the vitreous chamber, however, they must be also able to contain the appropriate dose to obtain effective concentrations over long periods of time. On the other hand, sustained-release antibody formulations have to maintain antibody stability and bioactivity during storage and release in vivo, which represents another relevant challenge to their development70. Therefore, although numerous intravitreal delivery systems are under research, most of them are in very early stages and only a few have been tested in clinical trials5. Intravitreal anti-VEGF drug delivery systems in development include hydrogels, liposomes, microparticles, nanoparticles, implants, and composite systems (i.e. combinations of two of the foregoing systems)71.
At the clinical level, implants are the only delivery systems that have been marketed for intravitreal administration. They are small solid devices that are surgically implanted or injected into the vitreous humor46. In Europe, commercial implants are currently available that provide the prolonged release of dexamethasone (Ozurdex®), fluocinolone (Iluvien®), and ganciclovir (Vitrasert® 4.5 mg, not marketed in Spain) (Table 3). However, there are no implants with anti-VEGF antibodies currently on the market71.
Some implants for the treatment of AMD are under research. The NT/503 cell line is loaded into an encapsulated-cell technology delivery system, implanted into the vitreous cavity every 12 weeks, and produces VEGF antagonists that are released into the retina. This device underwent phase I/II clinical trials: however, although the procedure seemed well tolerated, the phase II trial was halted due to lack of reproducible long-term efficacy72. We also draw attention to the Port Delivery System, which is a permanent and reusable drug reservoir that is surgically implanted through an incision in the sclera. It is loaded with ranibizumab solution 20 µL (100 mg/mL) that is released into the vitreous through a semipermeable membrane by passive diffusion. The device carries a customized needle that cleans the device and refills it with ranibizumab. This device is currently being tested in the ARCHWAY phase-III clinical trial (NCT03677934)73.
Periocular administration: subconjunctival, suprachoroidal, and transscleral routes
Periocular administration routes deliver drugs into the periocular tissues, producing local effects or providing access to intraocular tissues. Periocular administration includes the peribulbar, posterior juxtascleral, and retrobulbar routes, which are generally used to administer anesthetics; and the subtenon, subconjunctival, suprachoroidal, and transscleral routes, which are commonly used to administer drugs to the posterior segment2 (Figure 1).
The subconjunctival route refers to the administration of an injection or an implant below the conjunctiva. In the case of injection, an initial accumulation of the drug is formed that acts as a depot that undergoes slow depletion. In this route, the drug avoids elimination by the conjunctival blood and lymphatic circulation and must cross the sclera and choroid to reach the retina74. A small incision in the conjunctiva must be made in order to deliver subconjunctival/episcleral implants. Surodex™ is a biodegradable anterior chamber insert approved in Singapore and China, among other countries75. It is made of poly(lactic-co-glycolic acid) (PLGA) that provides the sustained release of dexamethasone for approximately 10 days for the treatment of inflammation after cataract surgery42.
Drugs administered through the suprachoroidal route slowly diffuse from the suprachoroidal space to the choroid and retina, minimizing systemic adverse effects and those related to the intravitreal route (endophthalmitis, retinal detachment, and cataract formation). Compared to subconjunctival injection, this administration route provides increased greater bioavailability due to its proximity to the retina and choroid15, does not interfere with the optical route, and is less invasive than the transscleral route2,76. Prolonged action is needed for this administration route to be attractive in a clinical setting. Controlled drug release formulations have been tested for suprachoroidal administration mainly in the form of implants, microneedles77, and micro/nano formulations to provide sustained release. All these approaches are still in preclinical and clinical phases78. Of these, we draw attention to the MicroPump®, a rechargeable nonbiodegradable implant comprising a microelectromechanical system that provides a constant release of a set volume of drug in the order of nanolitres76. Several phase-III clinical trials are currently underway of the suprachoroidal injection of triamcinolone acetonide in combination with various anti-VEGF agents for the treatment of AMD and posterior uveitis (NCT03203447, NCT02980874, NCT01789320)46.
The transscleral route bypasses the anterior segment barrier. Drugs administered through this route encounter the barriers of the sclera, choroidal blood flow, and retinal pigment epithelium. The transscleral route is less invasive and safer than the intravitreal route, but provides less intraocular bioavailability. This is because the removal path of the vitreous chamber moves outward while the drug moves inward (against the natural flow). These drawbacks can be overcome by modifications to the formulation2,79. Implants and iontophoretic devices are being developed to address this issue. Transscleral iontophoresis is a less invasive and well-tolerated method that allows drugs to be actively administered through the sclera. A mild electric current is used to allow the diffusion of the active ingredient from the reservoir to the site of action (retina/choroid). Within this approach we highlight the Visulex® iontophoretic device, which can release different corticosteroids via mild electrical currents. It has undergone a phase I/II clinical trial (NCT02309385) for the treatment of anterior uveitis. The success of iontophoresis-mediated drug delivery depends on several factors, such as charge density of the intended molecule, electric current applied, the duration of treatment application, and position of electrode placement46.
Systemic administration
Systemically administered drugs are distributed at the ocular level mainly in the choroid, iris, ciliary body, and retina. Specifically, the choroid receives around 85% of the ocular blood flow and has fenestrated blood vessels, allowing good extravascular distribution of drugs at this level. However, the passage of drugs from the choroid to the retina is obstructed by the pigment epithelial barrier80. In addition, the walls of the blood vessels of the iris and retina have very tight junctions between the endothelial cells, which slow down drug penetration. Consequently, small lipophilic compounds administered systemically can cross blood-ocular barriers, reaching and distributing to eye tissues, whereas the penetration of hydrophilic compounds and large molecules is highly restricted81.
In certain situations, systemic drug administration can be used to treat certain ocular pathologies. For example, antimicrobials can be administered for the treatment of endophthalmitis. Specifically, levofloxacin, moxifloxacin, linezolid, and meropenem are the best-documented agents that achieve therapeutic levels in the vitreous and penetrate to the site of action53,82. In addition, oral acetazolamide is administered for the treatment of glaucoma, systemic corticosteroids and anti-TNF antibodies for uveitis, and intravenous mannitol infusions for ocular hypertension. It is often the case that high doses of these drugs need to be administered such that they reach the ocular tissues in sufficient concentrations to exert the desired pharmacological effect. As a consequence, systemic adverse effects may appear81.
Verteporfin (Visudyne®) is currently available on the market for the treatment of exudative or wet AMD or systemic pathological myopia. This drug is administered by intravenous infusion and must be activated by light83.
The passage of substances from the bloodstream to the ocular tissues implies that they are sometimes exposed to systemically administered drugs that are not used for the treatment of ocular disease. This circumstance may lead to adverse effects at the ocular level, especially in highly polymedicated elderly patients. Drugs that cause toxicity at the retinal level after systemic administration include chloroquine, sildenafil, chlorpromazine, carmustine, and vigabatrin. Most of these adverse effects are relatively rare and transient under acute drug administration, but they can become more severe and frequent under chronic or long-term administration81,84.
Conclusions
Ocular drug delivery remains a major challenge for ophthalmology. Traditional formulations offer short drug residence times in the site of action, leading to the need for frequent administration, which directly affects therapeutic adherence and ultimately treatment success. Over the last few decades, some improvements have become commercially available, such as the use of emulsions, suspensions, and ointments, although none of these delivery systems achieve sufficiently long residence times. Recent studies have addressed new formulation techniques, such as the use of nanocarriers, medicated contact lenses, and implant/insert-type devices. Despite this activity, only a small number of these administration systems have been marketed for the treatment of diseases that affect the anterior chamber or the posterior chamber. For this reason, it remains relevant to continue investing in research on the development of new therapeutic strategies in order to improve the treatment and quality of life of patients and their use in routine clinical practice.